The antenna is a pivotal component in the design of any wireless system, playing a crucial role in facilitating wireless communication. The antennas for sixth generation (6G) and future communication systems are expected to be highly directional, have ultra-wide bandwidth and be efficiently reconfigurable. To meet these evolving requirements, diverse antenna designs have been conceptualized and proposed. The sub-THz (100 to 300 GHz) and THz (0.1 to 10 THz) spectra have been identified for 6G and future communication systems. Such high frequencies are characterized by high path loss and high atmospheric absorption. This article delineates the prerequisites of antennas for beyond 5G applications, addresses associated design challenges, surveys various antenna types suitable for 6G and future communication systems and provides a comprehensive guide for selecting antennas tailored to specific use cases such as ubiquitous Mobile Broadband (uMBB) and ultra-Reliable and Low-Latency Communications uRLLC, spectra (including mmWave and Terahertz) and enabling technologies. Three prominent types of antennas receive particular attention: patch antennas, extremely massive Multiple-Input-Multiple-Output (emMIMO) antennas and reconfigurable metamaterial antennas.
By 2030, the wireless connectivity landscape will comprise over 90 billion interconnected devices.1 This surge in connectivity is driven by emerging use cases, such as holographic presence, tactile internet and the demand for ubiquitous connectivity, including extremely high-definition video applications. These transformative paradigms necessitate unprecedented attributes such as vast bandwidth, elevated data traffic handling capabilities, remarkably low latency and heightened reliability.2, 3
At the core of these connected devices lies the antenna, which plays a fundamental role in wireless communication. All electronic communication systems and wireless networking equipment have antennas or elements that serve as antennas for the transmission and reception of electromagnetic signals.4 The antenna that will meet 6G and future communication system requirements is expected to be highly directional, intelligently reconfigurable, have ultra-wide bandwidth and be highly efficient.
This article provides a comprehensive exploration of 6G, delineates the key antenna performance requirements for 6G and future communication scenarios, reviews various antenna types suitable for these contexts and outlines prospective directions in the ongoing design and development of antennas for future communication systems.
THE ROAD TO 6G
To enable voice services, the first generation of mobile communication, 1G, was introduced in the 1980s, offering a data rate of 2.4 kbps. However, owing to its analog transmission nature, 1G suffered from limitations such as poor capacity, inconsistent delivery and inadequate security. Addressing these issues, second-generation (2G) networks emerged in the 1990s, leveraging digital modulation technology. With a data rate of 64 kbps on the Global System for Mobile Communications (GSM), 2G not only facilitated traditional voice communication but also introduced encrypted data services like Short Message Services (SMS). Both 1G and 2G systems were rooted in the public switched telephone network (PSTN).5
The third generation (3G) of mobile communications networks was developed around 2000 in response to the growing demand for various multi-data services, including internet browsing and video conversations. Code Division Multiple Access (CDMA) and Frequency-Division Multiple Access (FDMA) were exploited to create worldwide interoperability for microwave access (WiMAX), wide-CDMA, CDMA-2000 and synchronous CDMA with time division (TD-SCDMA) to meet the growing demand.6 3G networks provide a data rate of up to 14 Mbps.7
The fourth generation (4G) mobile communication standard was introduced in 2009. Known as Long-Term Evolution (LTE), it used both Frequency-Division Duplex (FDD) and Time-Division Duplex (TDD) modes. Orthogonal Frequency-Division Multiplexing (OFDM) technology in 4G enhanced data rates and spatial efficiency compared to earlier technologies. As data rates continued to rise, Multiple-Input-Multiple-Output (MIMO) and Coordinated Multiple Transmission/Reception (CoMP) technologies were introduced to support higher data rates, larger MBB connections and increased transmission bandwidth. The LTE mobile communication standard was upgraded to LTE-Advanced in 2011, allowing the use of unlicensed spectrum.7, 8
Current fifth-generation (5G) technology, employing non-orthogonal multiple access (NOMA), achieves nearly 100 Gbps data rates. However, future 6G networks are envisioned to use AI-driven MIMO-OFDM transceivers, potentially delivering up to 1 Tbps data rates. 5G networks deploy massive MIMO (mMIMO) and Beam Division Multiple Access (BDMA) to enhance system capacity, with BDMA enabling the allocation of orthogonal beams to users based on their locations.9
Recognizing the limitations of existing wireless communication technologies in handling the surge in data traffic and new applications, 6G networks are now emerging as the next frontier. These networks aim to meet evolving use cases and scenarios, promising ultra-high reliable data speeds with extremely low latency, high efficiency and ubiquitous connectivity.3, 6, 10 Table I provides a summary of the key features of mobile communication from 1 to 6G.
TABLE I - KEY FEATURES OF 1 THROUGH 6G COMMUNICATION TECHNOLOGY
6G KPIs
6G networks aim to provide ubiquitous connectivity, ultra-high data rates (1 Tbps), ultra-low latency (<1 ms), ultra-high reliability, ultra-high bandwidth, enhanced security and intelligent communication (see Figure 1). The values of these key performance indicators (KPIs) can be obtained from Alqahtani.11
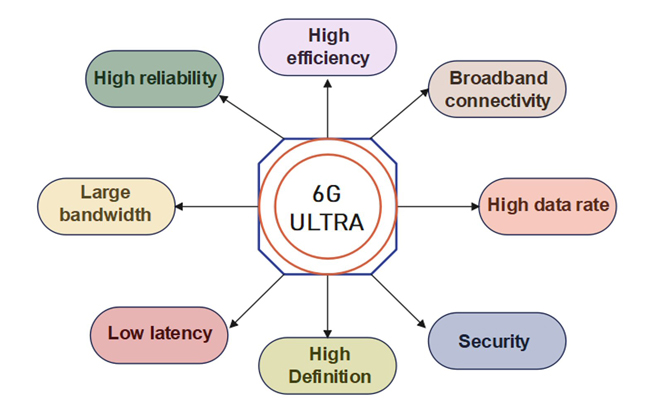
Figure 1 6G KPIs.
New disruptive use cases for 6G (see Figure 2) will lead to enormous bandwidth and hence, more energy consumption.2 To reduce energy consumption and increase cloud radio access, efficient network planning and allocation schemes have been proposed. 6G is expected to provide ubiquitous connectivity. This will be achieved by combining terrestrial and satellite communication, uncrewed aerial vehicles (UAVs), high-altitude platforms (HAPs), and heterogeneous networks. 6G communications systems will provide ultra-reliable and highly secured data transmission. To achieve this, it will use AI, blockchain technology and quantum communication to provide ultra-secure and reliable connections.
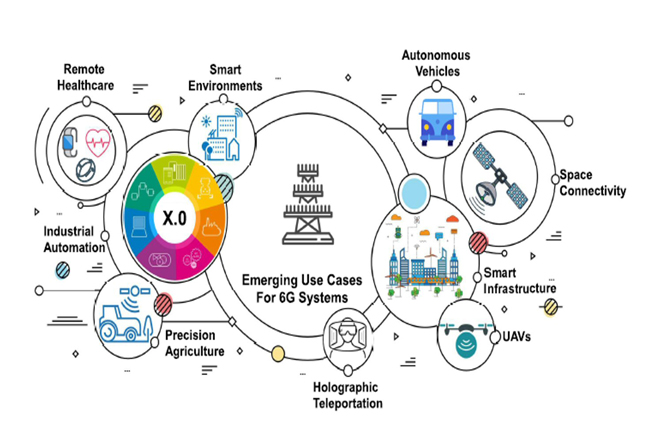
Figure 2 6G. disruptive use cases.3
There are three major drivers of 6G development: the explosive increase in mobile traffic, novel use scenarios and disruptive use cases (see Figure 3). It is expected that the number of machine-to-machine (M2M) connections will be over 90 billion by 2030.1, 12 There is now high demand for extremely high-definition video applications, enhanced screen resolution, M2M communications, vehicle-to-vehicle (V2V) communication and mobile-cloud services. 5G will not be able to effectively serve these needs. The features of 6G will enable its use in these applications and others such as holographic type communication (HTC), extended reality, global ubiquitous connectivity, intelligent transport, telemedicine and tactile internet.13, 14
5G was initially developed to meet three service scenarios: massive machine-type communications (mMTC), uRLLC and enhanced MBB (eMBB). The technical needs of future communication use cases (see Figure 2) cannot be satisfied by these 5G service scenarios. New use cases for 6G include uMBB (the availability of mobile communication all over the earth's surface), and massive URLLC (mURLLC).15
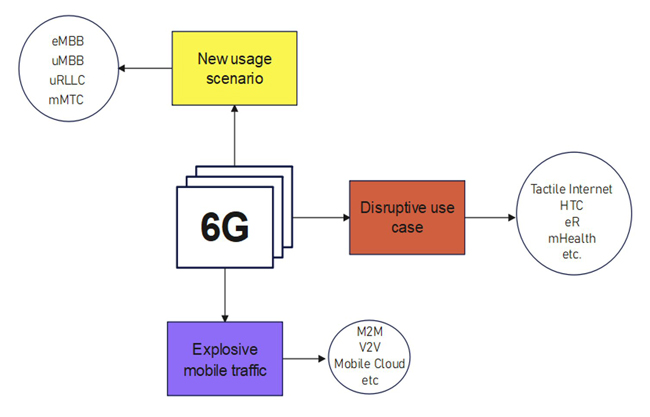
Figure 3 6G technology drivers.
6G ENABLERS
6G enablers and new technologies are shown in Figure 4 and listed in Table II. Emerging wireless technologies will rely on new air interfaces, new spectrum, a new paradigm, new networking and a new architecture to deliver ultra-reliable data transfer.
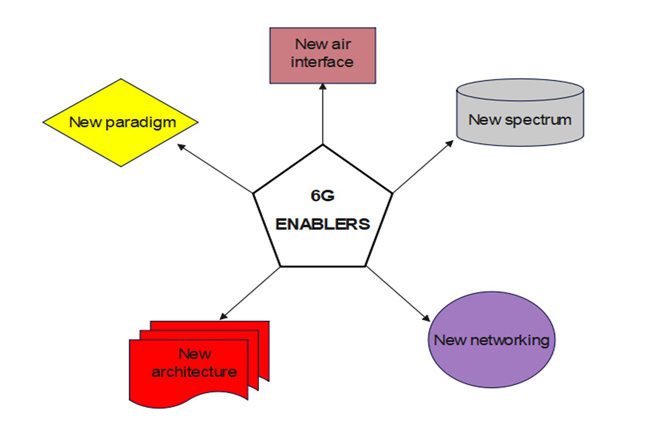
Figure 4 6G enablers.
New Air Interface
Active MIMO antenna arrays have been widely deployed in 4 and 5G. However, fast fading, high propagation loss, low diffraction and low non-line of sight path diversity16 are challenges that are faced as we move toward extremely high frequencies. 6G and future communication will go from small MIMO and active antennas to mMIMO and reconfigurable intelligent surfaces.17 These new air interfaces are expected to enhance system capacity, spectral/spatial efficiency and multiplexing gain while allowing incoming electromagnetic waves to be reflected in the desired direction.
New Spectrum
With an increasing number of connected devices, the widely used mmWave spectrum will be insufficient to accommodate new usage scenarios. Future communication technologies are expected to address wide bandwidth and high data rate needs through the use of the THz spectrum (0.1 to 10 THz),18 visible light communication (VLC) (400 to 800 THz) and optical wireless communication (OWC).19 Much work is ongoing in defining the use parameters, challenges and deployment scenarios associated with the new spectra.
New Paradigm
One of the most important 6G enablers will be AI.20 It is foreseen that 6G will shift from current conventional computing to a massive computer that integrates processing, storage, sensing, distributed communication and resource control to provide pervasive computing services.21, 22 AI, edge computing, blockchain and digital twins will enable intelligent reconfiguration of the network architecture, providing a secure end-to-end transfer of data and the efficient use of network resources.23
New Networking
The 5G core network currently deployed will not meet the internet work demand of future communication systems. Software-defined radio (SDR) and network function virtualization (NFV)24 have been identified to improve the radio access network (RAN) of 6G and beyond, while RAN slicing25 and open-RAN26 using software-defined networking (SDN) and network function virtualization (NFV) are potential ways of providing centralized and efficient network resource allocation. However, the increased number of virtual networks, placement of SDR controllers and traffic management are concerns.27
New Architecture
The network technologies of the past and those in use today were created to enable two-dimensional (2D) connections between network access points and user devices located on the ground. However, in 6G and future communication systems, an integrated space and terrestrial network (ISTN) is envisioned. An ISTN consists of three layers: the airborne layer (powered by UAVs and HAPs), the spaceborne layer (implemented by satellites) and the ground-based layer (terrestrial base stations).28 These layers will work collaboratively to realize the ubiquitous connectivity promise of 6G and future communication systems.
TABLE II - FUTURE COMMUNICATION-ENABLING TECHNOLOGIES

ANTENNAS FOR 6G AND FUTURE COMMUNICATION SYSTEMS
Driven by the data-centric and large bandwidth requirement of 6G, the mmWave frequency range from 30 to 300 GHz and the THz frequency range from 0.1 to 10 THz) will be used for 6G and beyond.30 Antennas to meet 6G and future communication system requirements are discussed in this section.