The IEEE 802.11ax standard, commonly referred to as Wi-Fi 6, was developed to support the ever-changing Wi-Fi traffic. For better communication efficiency with limited frequency resources, it is designed to handle multiple simultaneous connections to one access point (AP), as well as opening bandwidth to address increased video use—with special attention to 4K video streaming—and substantially more voice traffic due to carrier offload. Implementation of Wi-Fi 6 will yield better spectral efficiency and use of the new 6 GHz band, known as Wi-Fi 6E, as 802.11ax enables far better coexistence schemes, traffic scheduling and higher performance in dense scenarios. It does pose design challenges for engineers, however, and affects the test environments used to verify performance.
The impact of Wi-Fi 6 is expected to be profound. Figure 1 shows the projected growth of the technology in mobile handsets, consumer products and other network elements. Most premium phone models, particularly 5G enabled, already have Wi-Fi 6 embedded. Its cooperative solution with 5G is one reason for the expected fast rise of Wi-Fi 6. Practically speaking, Wi-Fi 6 is a local area context, and 5G is wide area. Wi-Fi 6 frees spectrum, as 5G use cases can be offloaded to use it.

Figure 1 Forecast Wi-Fi 6 IC shipments. Source: Omdia.
Wi-Fi 6 ENHANCEMENTS
To understand the increased testing requirements for Wi-Fi 6, we will first describe the changes in the 802.11ax standard that are providing these improvements.
SCHEDULING
Wi-Fi 6 is optimized to increase the average throughput by 4x for users in dense and congested environments. To achieve this enhanced throughput, Wi-Fi 6 can schedule down to 2 MHz bandwidth slices, a 10x improvement over the previous contention-based generations with 20 MHz bandwidth slices. The narrower slices create a dramatic drop in the noise floor by 8 dB, resulting in greater signal range or 8 dB more tolerance to noise and interference. This also increases efficiency by narrowing the subcarrier frequency spacing from 312.5 to 78.125 kHz.
Scheduling the 2 MHz slices extends battery life, as does the Wi-Fi 6 feature of target wake time (TWT). TWT enables a Wi-Fi 6 AP to trigger a client device to wake up and use battery power only when it’s needed. Wi-Fi 6 also makes better use of time and frequency resources, modulation, coding and spatial streams to minimize contention and improve capacity.
OFDMA/QAM INTEGRATION
Wi-Fi 6 uses orthogonal frequency-division multiple access (OFDMA), similar to the modulation used in 5G and LTE. OFDMA enables multiple stations to be served simultaneously, which improves capacity, latency and efficiency in dense signal environments. Wi-Fi 6 uses up to 1024 QAM modulation compared to 256 QAM in previous generations, increasing the peak data rate for a single user by 25 percent. This creates device design challenges, however; better modulation accuracy and more dynamic range for over-the-air (OTA) testing.
OFDMA is a new extension to multi-user MIMO (MU-MIMO) to increase the speed of Wi-Fi 6. MU-MIMO combines signals from separate antennas and spatial path differences to provide up to 12 spatial streams (eight in the 5 or 6 GHz bands and four in the 2.4 GHz bands), a considerable upgrade from the four provided by Wi-Fi 5. In beamforming mode, an AP can direct signals to desired clients and aim the effective receive (Rx) signal power away from other devices.
NEW PDDU FORMATS
At the PHY layer, 802.11ax distinguishes itself from legacy frames with four new packet protocol data unit (PPDU) formats: high efficiency, single user (HE SU), HE extended range (HE_EXT_SU), multi-user (HE MU) and HE trigger-based (HE_Trig).
Multi-user uplink (UL) transmissions typically require devices to be carefully calibrated to meet stringent power and measurement accuracy requirements. The 802.11 specification, however, allows device implementations with a wide range of capabilities. While the requirement for every device to support all PPDU formats has not been determined, every device must process the preamble for all transmission modes.
TRI-BAND ARCHITECTURE
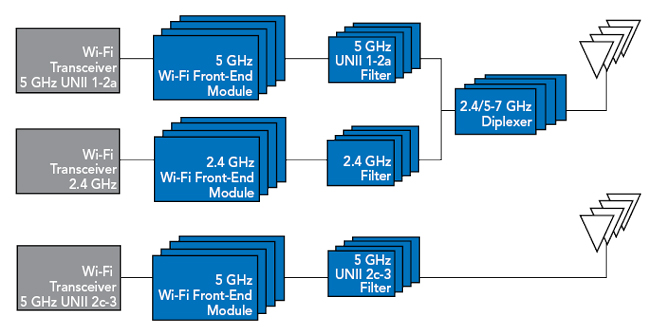
Figure 2 Wi-Fi 6 tri-band architecture.
A new tri-band architecture is used in Wi-Fi 6 designs (see Figure 2). The design requires advanced filtering to split the 5 and 2.4 GHz streams, which are divided into sets of three. Duplexing is necessary to reuse the advanced capabilities of the antennas to support the transmission of 2.4 and 5 GHz. The new architecture uses thermal efficiency isolation to compensate for the close spacing of active and passive components on the PCB, with advanced packaging required to maximize the thermal conductivity and minimize size.
Wi-Fi 6E EXTENSION
With the adoption of the Wi-Fi 6E (Extended) standard, the Wi-Fi Alliance expanded the spectrum to 7.125 GHz, enabling the 6 GHz band to also be used for unlicensed Wi-Fi operation. In conjunction with the tri-band architecture, Wi-Fi 6E establishes dedicated client communications and backhaul spectrum. Because of this, there is no longer a need to split the 5 GHz spectrum. The 6 GHz band can be dedicated to backhaul, creating an almost greenfield environment; i.e., it does not have to be compatible with legacy standards.
TEST REQUIREMENTS
The technology improvements of Wi-Fi 6 do more than enhance performance; they change the way products must be verified and the configuration of test systems. Instruments must measure to tighter parameters with tests performed OTA. New test items and complex measurement methods are being added to the IEEE 802.11ax standard, with the antenna as a key evaluation element for the 6 GHz band, because the antenna performance requirements change with frequency.
The European Telecommunications Standards Institute (ETSI) originally specified using a spectrum analyzer to conduct key performance measurements such as output power, spurious and occupied bandwidth. New specifications (ETSI EN 300.238 for 2.4 GHz and ETSI EN 301.893 for 5 GHz) require a Wi-Fi call box in the test system for regulatory testing to support the receiver blocking test. This test configuration is superior to using a non-signaling tester or AP to control the minimum data rate. The earlier approaches make it more complicated to control devices designed with several chipsets to the minimum data rate, because proprietary software is required for each chipset.
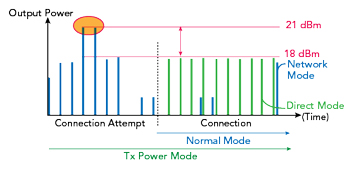
Figure 3 Call disconnect caused by excessive Tx power.
In addition to regulatory testing, mobile operators require power level tests during call connection to ensure there is no excessive output power that can lead to a disconnect. As this can occur in some cases, a useful capability is to measure transmit (Tx) power before call connection to find devices with excessive output power not caught by the IEEE procedure (see Figure 3).