Hypersonic missiles have risen to the forefront of conversations about global weapons arsenals. Attaining hypersonic speed is not new, with V2-powered missiles achieving hypersonic velocities in the late 1940s, and Russia’s Sputnik 1 satellite reaching space orbit in 1957. What is new and responsible for elevated interest and concern comes from a series of recent events.
The world took notice when, in 2021, reports surfaced of a Chinese nuclear-capable hypersonic missile circumnavigating the globe before impacting near its target. The missile missed the target by 24 miles according to reports. What is most interesting, though, is these reports did not become public until several months after launch, indicating the Chinese missile was undetected during its flight. In the ongoing Russia-Ukraine war, reports have Russia deploying at least one of their Kinzhal hypersonic missiles in Ukraine toward the end of March 2022. In early April, The U.S. Defense Advanced Research Projects Agency (DARPA) announced the second successful test flight of its Hypersonic Air-Breathing Weapon Concept missile.
Hypersonic weapons technology ushers in a new era of disruption and destabilization. As technologists solve the missile challenges and the offensive technology evolves, defensive systems must keep pace. A significant imbalance between the offensive and defensive capabilities of a new weapons technology could easily upset the uneasy deterrence among nuclear countries.
This article will explore the technology, challenges, spending forecasts and electronics opportunities for hypersonic weapons.
MACH 5

Figure 1 Scramjet engine concept. Source: NASA

Figure 2 Chinese DF-17 hypersonic glide vehicle. Source: nationalinterst.org
The term “hypersonic” is a bit imprecise. General agreement defines hypersonic velocity as the speed where air molecules start to break apart and ionize. This happens over a range of speeds and will vary depending on the airframe design, so the accepted convention has become to define hypersonic velocity as a minimum of Mach 5 (~3836 mph).
Hypersonic weapons developments fall into two categories: hypersonic cruise missiles (HCM) and hypersonic glide vehicles (HGV). HCMs derive their thrust to propel past Mach 5 from an air-breathing supersonic-combustion ramjet engine—a “scramjet.” Conventional ramjet engines use the forward speed of the vehicle to generate thrust. As the vehicle surpasses the speed of sound at Mach 1, the leading edges produce shock waves that slow the incoming air into the engine. In a ramjet engine, the intake passage compresses and ignites the incoming air to produce thrust. Current thinking has ramjet engines capable of reaching hypersonic speeds to Mach 6; however, at these speeds, the losses associated with the shock waves and the temperature increases from compressing the air make the technique very inefficient producing net thrust.
In the 1960s, NASA began addressing this challenge. Their solution was simple: compress the incoming air for subsequent combustion at higher speeds than a ramjet engine. While this was conceptually elegant and simple, severe design, material and coating challenges had to be solved to bring the idea to fruition. Implementation took four decades until the X-43A successfully demonstrated scramjet propulsion in March 2004. Figure 1 shows the basis for a supersonic-combustion ramjet. The design minimizes losses associated with slowing the air flow, so the engine can produce the net thrust required for a hypersonic vehicle. This concept has become the basis for engines working at speeds from Mach 5 to Mach 15. The engines still require air, which limits their theoretical altitude to the Kármán line at the edge of space, some 330,000 ft. above sea level. From a practical standpoint, HCMs have an altitude limit of ~100,000 ft.
HGVs are based on a different approach. Conventional rocket boosters launch these vehicles to a predetermined altitude, often just outside the atmosphere. Once the booster reaches this altitude, the HGV separates from the booster and the HGV begins picking up momentum and kinetic energy as it re-enters the atmosphere and glides to a target. During this glide phase, an HGV will use aerodynamic forces and control surfaces or small thrusters to “surf” the atmosphere between the Kármán line and altitudes of ~120,000 ft. Figure 2 shows China’s DF-17 hypersonic glide vehicle during one of that country’s military parades.
THE HYPERSONIC THREAT
Understanding the rudimentary theory of hypersonic weapons outlines the threat. Even though HCMs have an engine, they will be part of the weapons arsenal of a fighter jet or the payload on a missile, which will get the weapon to hypersonic speed more quickly. While either type of hypersonic vehicle can rely on simple kinetic energy as its destructive payload, more likely a hypersonic weapon will include a conventional or nuclear warhead. These weapons have flexible mission profiles, with either type capable of launching from and targeting air, land or sea-based platforms. This gives them the ability to target high value targets in any domain. In particular, the U.S. battle philosophy relies on aircraft carriers and their accompanying battle groups to establish naval superiority; hypersonic weapons present a big threat to those large, high value targets.
It is worth noting that the threat of hypersonic vehicles goes beyond their speed, operating altitude and ability to carry nuclear weapons. The world has coexisted with the threat of intercontinental ballistic missiles (ICBM) since the late 1950s. These missiles can carry nuclear warheads with velocities more than Mach 15, meaning a missile can reach any target on the globe within 30 minutes. The threat of hypersonic weapons stems from their attack profile (see Figure 3) and the difficulty detecting them.

Figure 3 Projectile trajectories and the likelihood of detection. Source: Plowshares.ca
Figure 3 shows the trajectory of a ballistic missile with a nuclear or a conventional payload, following the parabolic black dashed line trajectory to its target. The ballistic missile may also carry an HGV as a payload, with the HGV’s trajectory shown by the solid red line. The diagram also shows a fighter jet firing an HCM, with the trajectory shown by the solid blue line. The large black dot represents the target, which has a radar with a cone of detection shown by the light gray triangle. The smaller, colored dots in the figure show where the radar would likely detect the incoming projectiles. With no over-the-horizon capability, detection range becomes a function of altitude: the radar detects the ICBM first, while the hypersonic vehicles get much closer to the target before the radar can detect them. While the diagram shows the HCM at a lower altitude than the HGV, which may typically be true, both vehicles are maneuverable and capable of overlapping mission altitudes and profiles.
Detection networks are usually more sophisticated than the simplified illustration in Figure 3; they incorporate over-the-horizon and space-based systems to enable early detection of missile launches. In addition, ballistic missiles have a parabolic trajectory that will be known as soon as sensors identify the missile signature. This means earlier warning than the diagram predicts, which helps maintain the stasis among the nuclear ICBM-equipped countries. Hypersonic vehicles threaten to disrupt this equilibrium because they follow unpredictable trajectories, compress the time between detection and decision-making and present the uncertainty of whether the payload is a conventional or nuclear warhead.
HYPERSONIC DEVELOPMENT CHALLENGES
While hypersonic weapons are a threat and pose challenges to conventional defense systems, their development must overcome several hurdles before the weapons become mainstays of defense arsenals around the globe. Among these challenges:
Heat: Both types of hypersonic weapons systems operate in the atmosphere, and this means friction. Industry experts have estimated that friction caused by air resistance can easily increase skin temperature to more than 2200°C. This is several hundred degrees higher than the melting point of many of the lightweight metals used in projectiles. The rate and breadth of deployment of these weapons will require developing lightweight, easily manufactured airframe materials. The heat generated by internal electronics adds to the thermal problem; the internal electronics need to be protected from the heat generated on the vehicle.
Maneuverability: A big differentiator for hypersonic weapons is their unpredictable path, making them harder to detect and track. Mission profiles for these weapons have them operating in contested environments, facing a range of hostile defense systems. Implementing course changes requires taking advantage of vehicle aerodynamics or using small onboard thrust engines. These course changes, however small, will place enormous forces on the control surfaces and the vehicle frame.
These two challenges, coupled with aerodynamics and integration, fall in the realm of mechanical and materials expertise. In addition to the challenges of designing and manufacturing a vehicle that can achieve hypersonic speed, maneuver and prove cost effective and dependable, there are other challenges that relate to the mission profile of the weapon. These added challenges, which fall within the capabilities of the electronics industry, include the following:
Communications: The threat of a hypersonic weapon relies on its speed and unpredictability. This means that the command, control and communications infrastructure must be fast enough to implement course modifications and targeting information with little signal latency. Earlier, we defined the term “hypersonic” to be the velocity at which air molecules begin to ionize. Ionization creates a plasma shield around the hypersonic vehicle, and this atmospheric dissociation disrupts GPS, communication and telemetry signals. This is a fundamental problem, but there are developments aimed at tuning receiving antennas to enhance incoming communications signals during hypersonic travel. The Chinese are reportedly investigating THz communication networks to solve this problem.
Navigation, Timing and Accuracy: These challenges are related to communications. The sensors and internal electronics are stressed by the speed and the mission profile of the hypersonic vehicle, with the added complication of accuracy. If the hypersonic weapon is carrying a nuclear warhead, the destructive blast radius may accommodate some targeting inaccuracy, although this is an area for improvement. Initially, the U.S. is developing hypersonic weapons carrying only kinetic or conventional payloads, increasing the importance of accurate targeting systems.
DETECTION
To this point, the discussion has been on the benefits and challenges of the hypersonic vehicle as an offensive weapon. For the world to remain in that slightly uneasy state of weapons détente, defensive capabilities must be roughly equivalent to the offensive capabilities. An imbalance increases the likelihood of a hypersonic weapons first strike.
While much of the development has been on solving the offensive weapons challenges, military planners and government defense agencies are not overlooking the importance of defensive capabilities. As Figure 3 illustrates, hypersonic weapons flying faster at lower altitudes with less predictable flight paths compress the time for the conventional identify/decide/execute kill-chain analysis of military engagements. In addition to less time, decision-makers face much larger consequences and repercussions because the detected hypersonic vehicle may be carrying a kinetic, conventional or nuclear payload. Another concern is that decreasing the time to evaluate an incoming projectile increases the likelihood of a decision that escalates the situation.
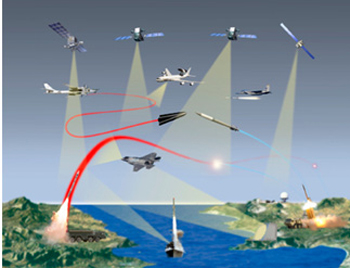
Figure 4 Integrated hypersonic defense network. Source: www.airforcemag.com/article/hypersonics-defense

Figure 5 Global hypersonic weapons spending forecast by country. Source: Strategy Analytics

Figure 6 Global hypersonic weapons spending forecast, RDT&E vs. procurement and support. Source: Strategy Analytics
In response, defense agencies are envisioning and developing defensive networks that incorporate and interconnect assets from the land, air, sea and space domains (see Figure 4). The figure shows two hypersonic weapons launched from a plane and a land-based launcher, shown by the red flight paths. The illustration shows various satellite, AWACs-type reconnaissance planes, fighter jets, ground- and ship-based radars identifying the launches, triggering interceptor missiles launched from ground, ship- and air-based assets. This multi-layered solution integrates space-based and ground-based sensor systems to identify weapons launches earlier in their flight paths.
The U.S. Department of Defense (DOD) recently announced it will begin evaluating the Long-Range Discrimination Radar homeland missile defense system for tracking intercontinental ballistic missiles and enabling next-generation threats like hypersonic weapons to be detected at longer ranges. The U.S. Missile Defense Agency (MDA) is moving forward with a system that will integrate into the Aegis Combat System on naval vessels. This system will use two space-based sensor systems to detect the launch of hypersonic threats, tracking them before they separate from their boosters.
In addition to conventional missile-based responses to hypersonics weapons, the defense community is developing a next-generation response. The MDA has selected several U.S. defense companies to develop an interceptor specifically designed to defeat hypersonic threats. The Glide Phase Interceptor program will develop a weapon to intercept and defeat hypersonic weapons in the glide phase of flight as they are maneuvering toward their targets. Several countries, including the U.S. and the U.K., are developing high-energy laser weapons to help defeat hypersonic weapons.
SPENDING FORECASTS
Not surprisingly, a credible new threat with the potential to disrupt the balance of power requires an in-kind development response. Figure 5 shows Strategy Analytics’ latest forecast for hypersonic weapons spending. We anticipate total spending on hypersonic weapons will reach nearly $22 billion in 2031 and believe the U.S. will be the largest spender, accounting for slightly more than the combined expenditures of China and Russia over the forecast period. The U.S. response reflects the size of its defense budget, but it also reflects a concern that U.S. hypersonic weapons development lags the efforts in China and Russia. These three countries will account for nearly 85 percent of total spending, with some development by other countries.
We have characterized hypersonic weapons as an old technology that has been repurposed to a new mission profile. The forecast in Figure 6 reflects the anticipated global spending profile. As with any new application, early funding is largely research, development, test and evaluation (RDT&E); until 2025, we expect more than 90 percent of funding will go toward development. Even though spending on RDT&E will peak in the middle of the forecast period and fall off, global defense agencies will still be spending more money on RDT&E for hypersonic weapons in 2031 than in 2021. As designers meet the challenges and the technology matures, we anticipate procurement spending on working hypersonic weapons will grow quickly. We expect militaries will spend slightly more than $13 billion on global procurement and support in 2031.
SUMMARY
This article has discussed the background, technology and development path for hypersonic weapons. Despite steady progress toward widespread deployment, there is a body of thought believing hypersonic weapons will prove too expensive and the technical challenges too great for them to be widely deployed. While this assessment may prove valid, the recent activities from China and Russia have demonstrated actual deployment and use of these weapons, highlighting the implications and threat of hypersonic technology.
Weapons using hypersonic technology have the potential to disrupt the uneasy global balance of power by tipping the scales toward countries that have hypersonic weapons capabilities. The anticipated speed, maneuverability and flexibility of hypersonic weapons strains the conventional decision loop by forcing a shorter command decision cycle. Since the same hypersonic glide vehicle or cruise missile can carry kinetic, conventional or nuclear payloads, these weapons also increase the risk of escalating a situation.
The stakes are high and, in response, defense agencies around the world are accelerating their offensive and defensive capabilities. We have identified $7 billion of hypersonic weapons expenditures in 2021, and we believe this will grow to nearly $22 billion in 2031. The scope and breadth of the development challenges mean that most of this activity is RDT&E, with the ecosystem for hypersonic weapons programs being government-funded programs awarded to large defense companies and research institutions, either alone or in teams.
While material and aerodynamic developments will be important, electronics and solid-state semiconductors will play a significant role in the success of hypersonic weapons. Communicating through the plasma shield created by hypersonic velocity becomes a critical need. Electronics must be robust to withstand high internal temperatures, while adding as little heat as possible to the ambient temperature. Networking capabilities in these extreme environments will become important if the mission requires multiple hypersonic weapons launches.
Early identification of hypersonic weapons is of paramount concern for defensive systems. This means longer range radars with increased linearity. Satellites will play an integral role in the detection network, leading to more sensitive, higher power sensors. The defense “network” will become a web, incorporating multiple domains and military branches, as we see with the U.S. DOD’s Joint All-Domain Command & Control program. Both offensive and defensive hypersonic development areas seem ripe for incorporating artificial intelligence and machine learning to reduce the time in the decision and command process.
Some have referred to the use of hypersonics weapons as a “Sputnik moment,” where a country falls behind or spends to catch up. The threat of this technology is too great to ignore, so we expect countries will spend to maintain their leads or to close the capabilities gap. As militaries commit to hypersonic weapons, future spending plans will create opportunities for electronics and compound semiconductors as militaries look to restore a global balance of power.