
Hybrid mode horn radiators with high aperture efficiency and low cross-polarization levels are commonly used in wideband or multi-beam antennas in modern telecommunications. Such applications usually require the accurate prediction and optimization of the antenna performance parameters for both fundamental and higher order mode operation. Although various analysis tools for the calculation of horn antennas are available, the synthesis and optimization of broadband, low cross-polarization and high directivity horns is still a challenge. To ease and accelerate the antenna design for engineers, Mician has developed a new horn antenna synthesis tool for multi-mode and tracking horns that supports many profile types, such as general corrugated, smooth wall or Potter horns.

The new synthesis tool utilizes body of revolution (BOR) elements, which are well suited to a specific design of circular and coaxial waveguide horns. This tool can either be used as standalone software or be plugged into the company’s μWave Wizard, where the existing Component Object Model (COM) capability of the software is utilized and extended to use the newly implemented features. The main window of the synthesis tool is used for basic definitions and the horn profile type, and for each different horn type a specific input window is provided to set up the geometry.
Individual windows are provided for the following horn types:
• Conical horns, dual-mode horns (Potter horns)
• Modified Potter horns
• Profiled smooth wall horns
• Corrugated horns with corrugation perpendicular to the horn axis
• Ring loaded corrugated horns
• Corrugated horns with axial corrugations
• Coaxial waveguide corrugated horns
• Hybrid geometry horns with axial and vertical corrugations
Figure 1 gives examples of these eight different types of horn antenna.
BOR Library Elements

A set of new elements has been developed for the radiation library of the μWave Wizard, which exploit the body of revolution symmetry of circular waveguide horn antennas. These elements coincide with the specifications for the various horn profiles. For example, there are elements for the (modified) Potter, the corrugated and the ring loaded horn profiles.
This makes the setup of the entire horn geometry much easier than previously, when the entire geometry had to be broken down into single circular waveguide and coaxial waveguide steps. The latter process is now carried out automatically inside the element.
Although the new set of BOR elements is calculated with the Mode-Matching (MM) method, the elements accelerate the computation for simultaneous analysis of fundamental and higher order mode excitations by subdividing the set of modes into de-coupled axial orders.
Additionally, these elements have been equipped with new types of output and calculation capabilities. Unlike other elements, where the electromagnetic fields are calculated only at one given position (for example, iris cross-section) and a single excitation (amplitude/phase), the field calculation inside the BOR elements comprises the entire structure and calculates the maximum over all incident phases. These calculated maximum fields can either be plotted along the horn profile or against the frequency by using the μWave graph tool provided by μWave Wizard.
Performance Parameters
The software is capable of calculating the following horn electrical performance parameters:
• Input return loss for the fundamental mode excitation and for the higher order modes (TMmn and TEmn) mode excitation
• Co-polar and cross-polar radiation patterns for the fundamental mode and for the higher order modes at any given distance from the radiator aperture
• Spherical Wave Expansion (SWE) parameters are optionally written to a data file either in μWave Wizard specific or in TICRA Grasp compatible format
• Phase center location, aperture efficiency, and 3 and 10 dB beamwidth are derived from the co-polar radiation patterns
• Electromagnetic fields and aperture field distribution along the horn
• Maximum field strengths versus frequency
• Ohmic losses
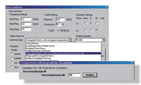
Synthesis
A typical design window for the synthesis of a horn antenna is shown in Figure 2. Initially, the user inserts general design parameters like frequency ranges and polarizations. Depending on the user’s demand and specifications (for example, cross-polar level, bandwidth) the type of horn is chosen. For most applications either a perpendicular corrugated horn profile or a Potter horn profile is sufficient to cover the specifications. Since closed form expressions exist for these horn types (and for the conical horn), the initial geometry is set up from a few additional design parameters (for example, maximum co-polar gain or beamwidth). In Figure 2 a gain of 20 dBi is specified for the corrugated horn antennas.
The initial definition of the frequency settings, horn profile type and the specification are input values of the closed form expression for a corrugated horn.1,2 The closed form results lead to initial values for all required geometries to generate a schematic of the corrugated horn. The values (see Figure 3) are assigned automatically to the project variables for the μWave Wizard schematics. Furthermore, all properties of the new BOR elements and the radiation module are specified by the direct link to the project variables.

The user can visualize the corrugated horn and begin an analysis of the horn antenna to calculate the input return loss response and the antenna patterns. The antenna pattern can be displayed as a 2D plot, a 2D-isoline plot and a 3D output plot. The typical computation time for one frequency step is about three seconds on a low end PC (Pentium Centrino). Besides the 3D output of the radiation pattern, a new feature enables the visualization of the field distribution along the longitudinal axis of the horn antenna. Figure 4 shows the results for a corrugated horn synthesized from closed form expressions.
The more experienced user also has the ability to overwrite the initial geometries given from the synthesis. These values are assigned as initial values to the μWave Wizard schematics of the corrugated horn and modification of the schematic variables provides users with maximum flexibility.
Optimization

The setup for optimization consists of two main parts: the defining of the optimization variables (degrees of freedom), including minimum and maximum values (constraints), and the defining of the goal functions (including the election of weight factors for various performance specifications). The geometry parameters from the synthesis are automatically set as optimization variables with an initial permissible variable range (usually ±10 percent).
At this stage the user controls these optimization variables and either fixes the values (for restrictive guidelines, for example, fixed input radius), redefines the constraints (for example, minimum gap widths) or sets up equations for a number of variables to limit the number of degrees of freedom for faster optimization. Figure 5 shows the specification of the goal function for an optimization.
The next step is the definition of the goal function and the selection of an optimizer, the maximum number of iterations and the maximum computation time during an optimization task. The goal function consists of various specifications over certain frequency ranges, to which individual weight factors are added. For basic specifications like telecom mode return loss, maximum co-polar and cross-polar gain, phase center variation, aperture efficiency and 3 or 10 dB beamwidth, there are easy to use pre-defined output variables. The inclusion of these variables into the goal function can be switched on or off in the synthesis tool. More advanced specifications can be defined by using these output variables in user-defined equations.
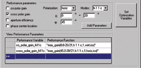
After the optimization setup is complete, the user starts the optimization. The user can monitor the process as the goal function is minimized, including partial errors for each optimization specification and the behavior of the variables in the desired range. The best result is stored automatically. Therefore, the user can interrupt the optimization at any stage, can change some settings (goal function weight factors or variable ranges) and then continue the optimization without losing the previous optimal result. If required, the user can also check the power handling performance during the optimization process by inserting a field calculation analysis. The field calculation inside the structure usually takes much longer than ordinary analysis, so it is not enabled by default.
If the results of the optimization are either within a given error limit, within the user-controlled maximum number of iterations or if the maximum computation time is reached, a final overall analysis is performed. For this the user normally adopts a slightly modified setup (for example, more frequencies, higher accuracy settings, more comprehensive output) compared to the analyses performed during optimization.
Validation
The radiation feature based on the spherical wave expansion is verified against measured radiation patterns.3,4 Apart from simple geometries (for example, radiating circular waveguide) this has been done for an optimized Potter horn, as shown in Figure 6. The measured co-polar and cross-polar patterns are also depicted in the figure. It should be noted that the results coincide with the original data (curve labeled ‘Theory’).

Conclusion
The new horn antenna synthesis tool provides many horn profiles for different kinds of horn antennas. The initial geometry given by a closed form expression is assigned directly to the element properties of the new BOR radiation elements. Built-in optimizers can be used to efficiently improve the initial performance to meet most stringent horn performance requirements. The new software is fully compatible with the general µWave Wizard software, which when combined optimizes the radiated antenna performance, including the feed chain RF response (even with manufacturing errors). This novel CAD tool provides exceptional design capabilities by yielding very accurate performance predictions. The output data in the form of SWE can then be used as input for general reflector antenna analysis.
Acknowledgment
This work has been funded by ESA/ESTEC under contract number 19986/06/NL/JA and was performed with the collaboration of MacDonald, Dettwiler and Associates Corp. (MDA). The company also wishes to thank Jarek Uher and Sylvain Richard from MDA and Pablo Sarasa from ESA/ESTEC for their help and guidance.
References
1. C. Granet, G.L. James, R. Bolton and G. Moorey, “A Smooth-walled Spline-profile Horn as an Alternative to the Corrugated Horn for Wideband Millimeter-wave Optimization,” IEEE Transactions on Antennas and Propagation, Vol. 52, No. 3, March 2004, pp. 848–834.
2. C. Granet and G.L. James, “Design of Corrugated Horns: A Primer,” IEEE Antennas and Propagation Magazine, Vol. 47, No. 2, April 2005, pp. 76–84.
3. C. Reiter, “Dielektrisch Ausgekleidete Rundhornstrahler,” PhD Thesis, University of Braunschweig, VDI, Series 21, No. 165, 1994.
4. J.M. Reiter, “Analytische Felddarstellung auf Nichtseparablen Oberflächen,” PhD Thesis, University of Bremen, VDI, Series 21, No. 180, 1995.
Mician GmbH
Bremen, Germany
+49 (421) 168 993 51
www.mician.com