Most end users require passing at least a Class 3 level of performance. The study reflected in this article confirms that the 555 to 960 MHz frequency band meets CISPR 25 standards, emphasizing the importance of these classes in maintaining compliance and system reliability. The 555 to 960 MHz frequency range, part of the broader 200 MHz to 1 GHz spectrum, requires a log-periodic antenna, like the example in Figure 2, for EM interference testing.
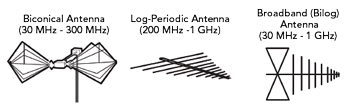
Figure 2 Log-periodic antenna.8
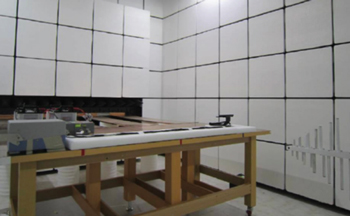
Figure 3 Radiation measurement setup showing log-periodic antenna.
When measuring specific frequency bands, the measurement environment9 has been designed and the antenna has been chosen in accordance with the CISPR 25 regulations. Figure 3 illustrates the setup concept for the measurement environment. Table 2 shows the frequency response and polarization capabilities of various antenna types. In this case, the measurement frequency band is 555 to 960 MHz and the log-periodic antenna is the best solution.
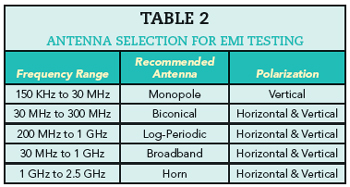
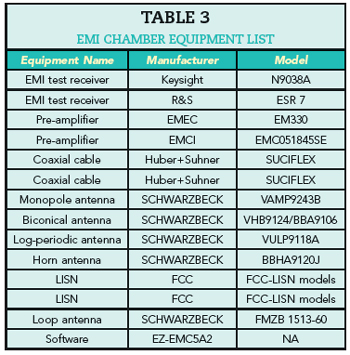
The antenna setup involves meticulous positioning and orienting the antenna to maximize testing accuracy. The setup process specifies the distance between the antenna and the equipment under test and details the frequency range the log-periodic antenna covers. Additionally, the usage scenarios for vertical and horizontal polarization are outlined, which may vary depending on the testing frequency range. Representative examples of all necessary measuring instruments, like spectrum analyzers and cables, are listed in Table 3 with guidance on their connections.
The test environment may also be described with specific requirements, such as anechoic or low-reflection chambers, to ensure consistent and comparable test results across different testing sites and conditions. It is important to note that this is a generalized explanation and the specifics can vary with different editions or revisions of the CISPR 25 standard. The setup outlined provides a reference standard for experimenters to ensure reliable testing outcomes.
EXPERIMENTAL RESULTS
In the radiation emission tests, spanning the frequency range of 555 to 960 MHz, values exceeding the limits were identified, particularly at specific frequencies. Notably, the average radiation levels at the 806.5 MHz frequency exceeded the prescribed limit by 2.51 dB and the measured result at 924 MHz was 0.69 dB higher than the standard. The graph of the results and tabulated data is shown in Figure 4.
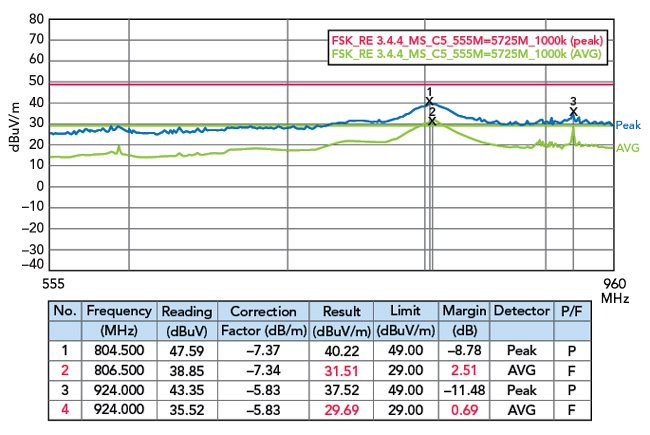
Figure 4 Radiation emissions results.
A particular focus has been placed on the 806.5 MHz frequency to address these non-compliance issues, where the deviation was most significant. Drawing from the stringent criteria outlined in Class 5, as outlined in Figure 1, both peak and average emission limits are defined at 49 dBμV/m and 29 dBμV/m, respectively. Any frequency exceeding these thresholds is considered a failure that necessitates remedial actions. Figure 1 delineates more lenient criteria under Class 3 and Class 4, with peak limits of 61 dB/m and 55 dB/m and average limits of 41 dB/m and 35 dB/m, respectively. The test details reveal that for average emissions, the 806.5 MHz frequency showed a reading of 31.51 dB/m, surpassing the limit by 2.51 dB and the 924 MHz frequency registered 29.69 dB/m, exceeding the limit by 0.69 dB. For peak emissions, the 804.5 MHz and 924 MHz frequencies measured 40.22 dB/m and 37.52 dB/m, respectively, well within the Class 5 peak limit, thereby not exceeding the stringent 49 dBμV/m limit.
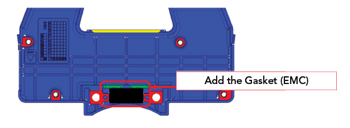
Figure 5 Identifying the problem area.
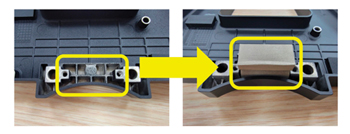
Figure 6 Implementing the conductive foam.
Testing circuit boards used in automotive electronic screens with a near-field probe detected excessive EM interference that could potentially affect screen function. The result showed notable spikes at specific frequencies, suggesting circuit instability. Despite recording peak values, the measurement equipment only indicated high noise levels without providing detailed insights, hinting at issues such as unstable power, inadequate grounding or component failure. Additional tests are needed to pinpoint the noise source and address it effectively.
Implementing the Conductive Foam Solution
The region indicated in Figure 5 was identified as the cause of the oscillations that pushed the measured results past the limits highlighted in Figure 4. This effect occurred after mounting the PCBA within the chassis. Before the assembly of the chassis, this specific area did not exhibit high noise levels. The conjecture is that the noise increase in this area could be due to the assembly of the chassis, which contains metal components.
To address this issue, foam encased in a conductive fabric was used to encapsulate the metal area. After this modification shown in Figure 6, subsequent measurements with a near-field probe showed an 8 dB reduction in noise. This demonstrates the effectiveness of employing conductive foam in reducing noise in areas prone to oscillation.
Initially, radiation at the 806.5 MHz frequency exceeded limits by 2.51 dB. Retesting after the foam was installed revealed an 8 dB improvement at the 790 MHz, significantly better than the original levels and confirming the foam’s effectiveness across multiple frequencies, including the initially problematic 806.5 MHz and 924 MHz. Despite all intervals passing upon retest, caution is advised due to values nearing failure thresholds. Notably, retests at 806.5 MHz and 924 MHz showed compliance with CISPR 25 standards, which prescribe limits for various types of EMI measurements to ensure the operational integrity of automotive components and that the components do not cause and will not suffer from interference. The retest results are shown in Figure 7.
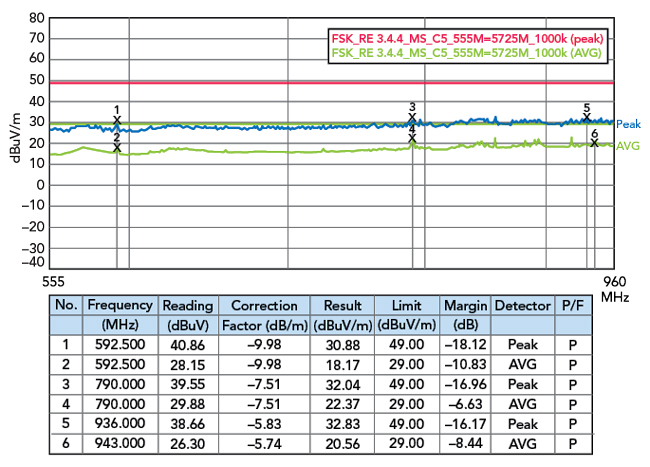
Figure 7 Radiation emissions results with the conductive foam.
CONCLUSION
This study identifies an issue with radiation exceeding the CISPR 25 standard limits in automotive electronic vehicle information system screens. The study pinpointed the PCBA used in the screens as the primary radiation source. A significant improvement was achieved by modifying a gasket with conductive foam, effectively reducing the radiation levels. The excess radiation presents a compliance threat that could prevent the product from legally entering the market, affecting both the development cycle and costs. The identification of the PCBA as the primary radiation source underscores the necessity for design improvements focused on this area.
However, the scope of the study is limited to the 555 to 960 MHz frequency range. It is specific to a particular model of automotive electronic screens, which may not be universally applicable across other models or brands. Future research should encompass a broader frequency range and investigate why the PCBA is a significant radiation source, considering more comprehensive improvement strategies. Additionally, evaluating other radiation reduction technologies or materials beyond conductive gasket foam and conducting experiments on a larger scale across various automotive electronic devices would expand the applicability of this study. This research lays the groundwork for addressing specific radiation issues, proposing using conductive gasket materials to shield against radiated emissions. While conductive foam installation offers an effective way to mitigate the issue, the exact source of emissions is unknown, suggesting further investigation is needed to ensure compliance with regulations.
References
- IEC, International Special Committee on Radio Interference (CISPR) Guidance for Users of the CISPR Standards, February 2015.
- UNECE, “Uniform Provisions Concerning the Approval of Vehicles with Regard to EM Compatibility,” Regulation No. 10.
- C. R. Paul, Introduction to EM Compatibility, 1st Ed. John Wiley Sons, 1992.
- M. P. Robinson, T. M. Benson, C. Christopoulos, J. F. Dawson, M. Ganley, A. C. Marvin, S. J. Porter and D. W. P. Thomas, “Analytical Formulation for the Shielding Effectiveness of Enclosures with Apertures,” IEEE Trans. Electromagn. Compat., Vol. 40, August 1998, pp. 240–248.
- M. P. Robinson, J. D. Turner, D. W. P. Thomas, J. F. Dawson, M. D. Ganley, A. C. Marvin, S. J. Porter, T. M. Benson and C. Christopoulos, “Shielding Effectiveness of a Rectangular Enclosure with a Rectangular Aperture,” Electronics Letters, Vol. 32, August 1996, pp. 1559–1560.
- C. F. M. Carobbi and D. Izzo,” Evaluation and Improvement of the Reproducibility of CISPR 25 ALSE Test Method,” IEEE Transactions on EM Compatibility, Vol. 60, No. 4, August 2018, pp. 1069-1077.
- C. Carobbi and D. Izzo,” Reproducibility of CISPR 25 ALSE Test Method,” IEEE International Symposium on EM Compatibility Signal/Power Integrity (EMCSI), 2017, pp. 57–62.
- T. Hegarty, “An Overview of Conducted EMI specifications for Power Supplies,” Texas Instruments, Tech. Rep., June 2018.
- CISPR, “Vehicles, Boats and Internal Combustion Engines–Radio Disturbance Characteristics–Limits and Methods of Measurement for the Protection of On-board Receivers,” CISPR, Tech. Rep., October 27, 2016.