
THz-capable isolators are looking to resurrect the “dead zone,” a region of the electromagnetic (EM) spectrum between what is commonly used for electronic and optical applications. This band, often called the “terahertz gap,” resides between 100 GHz and 30 THz. Recently, IMRA, a research and innovation organization that develops essential technologies for industrial use, overcame many of the issues associated with the THz regime when working at frequencies above 300 GHz.
Researchers at the Colorado-based laboratory were looking to use molecules as stable frequency references that could potentially be used to develop molecular clocks. During their research of alternatives to the current state-of-the-art optical atomic clocks operating at several hundreds of terahertz,1 IMRA researchers encountered the common issue of standing waves, also referred to as signal reflections or mismatches. These undesirable waves, or ripples, can attenuate power output, distort the information on the carrier and, in extreme cases, damage internal components.

Figure 1 26.5 to 400 GHz isolators.
Engineers rely on Faraday rotation isolators, more commonly referred to as isolators, to alleviate the problem of standing waves at lower microwave frequencies. An isolator is a two-port component that allows EM signals to pass in one direction but get absorbed in the opposite direction. The challenge is that traditional isolators fail to deliver at higher frequencies within the THz regime.
Under a NASA-awarded contract, Micro Harmonics Corporation (MHC) produced a line of mmWave isolators capable of operating between WR-28 and WR-2.8 (26.5 to 400 GHz). Examples of these devices are shown in Figure 1.
These commercial-off-the-shelf components had to be completely redesigned to deliver in five critical categories:
ISOLATION
The distance between components in mmWave systems often exceeds a wavelength, causing reflected signals to be out of phase. This can perturb the operating point of the upstream component. Sweeping frequencies causes phase changes that create nulls, dips and degraded performance. An isolator inserted between components will absorb the reflected signal and the problem will disappear.
Isolation measures how much of the signal traveling in the reverse direction passes through the isolator. The highest possible isolation occurs when the reverse wave is rotated exactly 45 degrees into the plane of the isolator’s resistive layer. Isolation can degrade by as much as 10 dB when the signal rotation is off by just 1 degree. MHC fully characterizes each isolator on a vector network analyzer rather than spot-checking at a couple of frequencies in the band to ensure total compliance.
INSERTION LOSS
While isolation is paramount, suppressing the reverse wave in an isolator cannot come at the expense of forward input signal attenuation. For traditional isolators, insertion loss is low in the microwave bands, but the loss becomes increasingly problematic at mmWave and THz frequencies. In the WR-10 band (75 to 110 GHz), the insertion loss can exceed 3 dB, meaning half of the signal power is lost. The loss in the WR-5.1 band (140 to 220 GHz) climbs to more than 5 dB. Because of high losses, traditional isolators were often precluded from use in mmWave systems.
Faraday rotation isolators use ferrite discs to rotate the signal. However, the traditional manufacturing method involves tuning the magnetic bias field of ferrites substantially longer than the minimum required length to achieve optimal performance. This delivers good isolation but at a much higher insertion loss.
To minimize loss, the goal is to reduce the ferrite length as much as possible. The MHC design saturates the ferrite with a strong magnetic bias field, enabling the ideal 45 degrees of rotation in the shortest possible ferrite length. This reduces insertion loss to less than 1 dB at 75 to 110 GHz and only 2 dB at 220 to 330 GHz.
LOW PORT REFLECTION
A good isolator must also have low port reflections. The importance of low port reflections is often overlooked. An isolator with high port reflections creates an alternate set of standing waves. The adjacent components are still adversely impacted by out-of-phase signals reflected into their ports. High isolation and low insertion loss are of little value if the port reflections are large. A good VSWR at mmWave frequencies is 1.5:1 or less.
HIGH POWER RATING
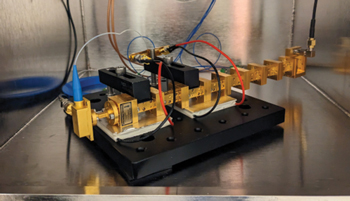
Figure 2 Terahertz oscillator developed by IMRA.
Power in the reverse signal is absorbed in the isolator, resulting in heat. Handling more heat requires a higher power rating. Historically, heat was not an issue with very little transmit power available at mmWave frequencies. However, as higher power sources become available at mmWave frequencies, the importance of power ratings increases.
To handle the problem of high heat loads, some newer isolators already incorporate diamond heat sinks into their design. Diamond is the ultimate thermal conductor, approaching 2200 W/m•K, more than 5x higher than copper. Diamond effectively channels heat from the resistive layer in the isolator to the metal waveguide block, lowering operating temperatures for improved reliability.
SMALL FOOTPRINT
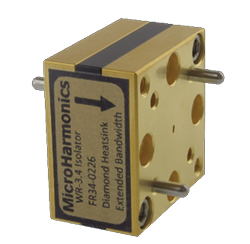
Figure 3 MHC WR-3.4 isolator.
Minimizing the size and weight of mmWave components is especially important in wireless applications. A standard traditional-style isolator in the WR-10 band is about 3 in. long, with a center cylindrical section diameter of about 1.3 in. However, the MHC design is rectangular and can be as small as 0.75 in. per side and 0.45 in. thick.
EXAMPLES
Building upon a firm understanding of the principles and the development of the components is allowing researchers and manufacturers to start to close the terahertz gap. Figure 2 shows an experimental DKS microcomb THz oscillator and spectroscopy of N2O. Figure 3 shows a Micro Harmonics WR-3.4 isolator operating in the 220 to 325 GHz band. With this isolator providing 30 dB of attenuation, IMRA researchers could talk to N2O molecules in the test setup of Figure 2.
Reference
- J. Greenberg, B. M. Heffernan and A. Rolland, “Terahertz Microcomb Oscillator Stabilized by Molecular Rotation,” APL Photonics, January 2024, Web: pubs.aip.org/aip/app/article/9/1/010802/3012375/Terahertz-microcomb-oscillator-stabilized-by.
Micro Harmonics
Fincastle, Va.
540.473.9983
MicroHarmonics.com