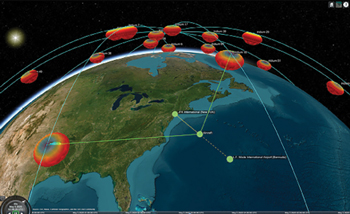
Figure 1 Rendition of a LEO satellite.
Interest and investment in commercial space satellite systems are booming. Private investors have injected more than $23.5 billion in private-sector funding into space-related companies since 2021.1 Tech giants like SpaceX and Amazon (Kuiper) have launched space initiatives to increase global broadband access. Historically, satellite communications (satcom) have been used for voice communication, defense and space exploration; however, the introduction and proliferation of low earth orbit (LEO) satellites have lowered the financial barrier required to launch satellites and opened the door for new use cases. This economic benefit is due to two factors. The first benefit relates to the size of the satellites; the latest Starlink LEOs from SpaceX are as small as a kitchen table. The second benefit revolves around the ability to launch multiple LEOs simultaneously, which helps control launch costs. However, while LEOs make satcom systems more economically viable, they introduce complexity and require engineers to manage higher Doppler shifts, interference and network complexities. A representative LEO satellite is shown in Figure 1.
TRENDS DRIVING SATCOM SYSTEMS ADOPTION
Ubiquitous connectivity, an environment where devices can create, share and process data from virtually anywhere, is one of the key trends driving the adoption of LEOs. Despite progress in building the terrestrial wireless communications infrastructure, significant portions of the world, such as rural communities and oceans, remain devoid of cellular connectivity due to cost or geography. Satellites are a critical enabling technology in the wireless industry’s work toward closing the connectivity gaps between urban and rural areas.
In addition to cellular accessibility, LEOs can also improve cellular capacity. Consider the following market data from Statista: there are currently 4.6 billion smartphone users worldwide2 and the number of Internet-connected devices is expected to reach more than 29 billion worldwide by 2030.3 More and more people are using the internet, increasing the global cellular system demand. Wireless companies continue to invest in terrestrial infrastructure, as commercial satellites have not always been cost-effective; however, the cost of LEOs is decreasing, making them a viable option to address the increasingly limited bandwidth, especially in remote areas.
Finally, disaster recovery communications are a key trend driving satcom adoption, as extreme weather events are becoming more powerful and frequent.4 Cellular infrastructure is frequently knocked out during these events, prompting satellite activation to ensure first responders, government officials and residents can broadcast and receive critical safety information. This use case was validated when Starlink positioned 120 satellites over Southwest Florida and other areas affected by Hurricane Ian5 when terrestrial cellular infrastructure was destroyed.
SIGNAL LATENCY AND POWER AMPLIFICATION
Before LEOs, satcom systems primarily used geostationary earth orbit (GEO) satellites. Three GEO satellites, properly spaced in longitude and revolving at the same rate as the rotation of Earth, can provide virtually full Earth coverage. Three GEO satellites can cover the planet with a few crosslinks but, unfortunately, are more expensive to build and launch than LEOs. Furthermore, the distance of GEO satellites from the ground and each other introduces latency in their signals. While GEO satellites are acceptable for email and other non-real-time communications, phone and video calls experience significant delays that impede natural communication.
Signal delays are much shorter with LEOs because they are closer to Earth’s surface. However, transmitters need more power to communicate with LEOs than terrestrial networks. This is because terrestrial network signals travel between 5 and 10 km, while LEO signals travel up to 2000 km and suffer more signal loss.
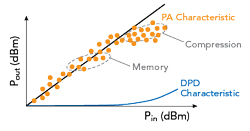
Figure 2 Power amplifier and DPD measured data.
The diminutive size of LEO satellites is both a boon and a design challenge. The LEO power amplifiers (PAs) must be physically small yet have enough power to transmit a signal to their intended target. In an ideal world, satellite engineers want PAs to have a linear characteristic even when driven with high-power inputs. However, when a PA is driven too hard, it will go into compression and that can significantly distort the signals. Figure 2 shows a representative PA characteristic illustrating the effects of both nonlinearity (compression) and memory.
One method to counteract and improve these distortions is to use digital predistortion (DPD) subsystems in the transmitter. DPD applies an “inverse PA” characteristic to the signal that causes the output signal of the PA to be more linear. DPD tools, such as those in the MathWorks Communications Toolbox®, are increasingly using AI to improve results. Figure 2 also shows the gain characteristics of a representative DPD circuit and it becomes clear how the DPD inverse characteristics help compensate for the amplifier nonlinearity.
RF LINKS, OPTICAL LINKS AND PHASED ARRAYS
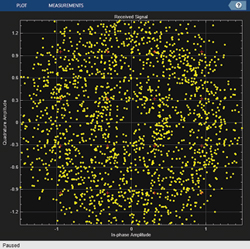
Figure 3 Representative received signal data.
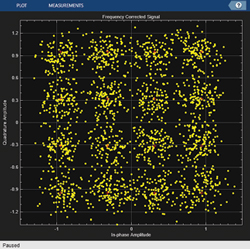
Figure 4 Frequency-corrected signal.
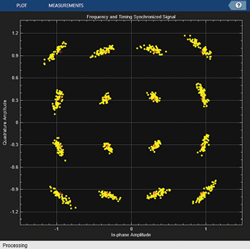
Figure 5 16-QAM constellation data with frequency and timing synchronization.
Interference also presents a challenge when using LEOs for satellite communications systems. The main reason for this is the simple fact that there are nearly 6000 LEOs in orbit currently.6 Traditional RF links have long been used in satcom systems, but engineers increasingly choose optical links when possible. Optical beam patterns are more narrow than traditional RF links, whose broad beams can spill over into other receivers and cause interference. Interference from optical systems is significantly reduced due to limited signal spreading.
Finally, satellite engineers can also use phased arrays, which are groups of computer-controlled antennas that create a beam that can be electronically steered to point in different directions. Phased arrays can spatially null out interference and direct energy at a particular spot on the ground. Phased array systems maximize beam energy in the direction of the signal of interest and insert beam nulls in the direction of interference, maximizing signal-to-interference plus noise ratio (SINR).
DOPPLER EFFECT AND FREQUENCY SHIFT
Unlike GEOs, LEOs do not revolve around Earth at the same rate as the planet’s rotation. This means that they are constantly moving either toward or away from receivers. This movement creates a Doppler effect that satellite engineers must manage. In engineering terms, the Doppler effect refers to the difference in frequency between the transmitted wave and the received wave due to transmitter or receiver motion. Doppler challenges require satellite engineers to acquire and track the continually changing center frequencies of LEO satellites.
The frequency and phase of the transmitter and receiver must be completely locked in to ensure the waveforms are successfully demodulated. However, large Doppler shifts cause the frequency, phase and timing to be out of sync. As a result, multiple closed loops must be implemented in these receivers to eliminate Doppler-induced frequency offsets. Synchronization must happen at the frame, symbol timing, carrier frequency and carrier phase levels. Figure 3 shows representative received I/Q data plotted on a constellation diagram and specific constellation points cannot be identified.
Figure 4 shows the constellation diagram with a frequency-corrected signal and while the constellation diagram is becoming clearer, the received signal can be only partially identified.
Figure 5 shows the constellation diagram when the timing and frequency have been synchronized and the signal aligns with the expected 16-QAM constellation points. Products from MathWorks can help designers model and understand these effects and how to successfully close the link for a satcom network.
CONCLUSION
Many satellite engineers use reference receiver designs from products such as MATLAB™ so they do not need to constantly reinvent the wheel. With minor customization from the reference designs, satellite engineers can design robust receivers that can operate in challenging RF environments.
LEOs have received their fair share of attention because of their compelling short- and long-term use cases. Companies like Apple are already tapping into satcom networks and that is only the start. As satcom continues influencing the wireless industry, engineers should familiarize themselves with the uses, challenges and enabling technologies for these satellite networks.
References
- “Space Tech Funding Comes Back Down To Earth,” Crunchbase, July 2023, Web: news.crunchbase.com/venture/space-tech-funding-drops-spacex-relativity.
- “Number of Smartphone Users Worldwide from 2013 to 2028,” Statista, August 2023, Web: www.statista.com/forecasts/1143723/smartphone-users-in-the-world.
- “Number of Internet of Things (IoT) Connected devices worldwide from 2019 to 2023, with Forecasts from 2022 to 2030,” Statista, July 2023, Web: www.statista.com/statistics/1183457/iot-connected-devices-worldwide.
- “Explaining Extreme Events from a Climate Perspective,” American Meteorological Society, Web: www.ametsoc.org/index.cfm/ams/publications/bulletin-of-the-american-meteorological-society-bams/explaining-extreme-events-from-a-climate-perspective/.
- “Elon Musk to Provide Florida with Starlink Satellites in Response to Hurricane Ian,” Reuters, October 2022, Web: www.reuters.com/world/us/elon-musk-provide-florida-with-starlink-satellites-response-hurricane-ian-2022-10-01.
- “UCS Satellite Database,” Union of Concerned Scientists, December 2005, Web: www.ucsusa.org/resources/satellite-database#:~:text=Total%20number%20of%20operating%20satellites%3A%206%2C718%20United%20States%3A,Russia%3A%20174%20China%3A%20590%20Other%3A%201%2C425%20LEO%3A%205%2C938.