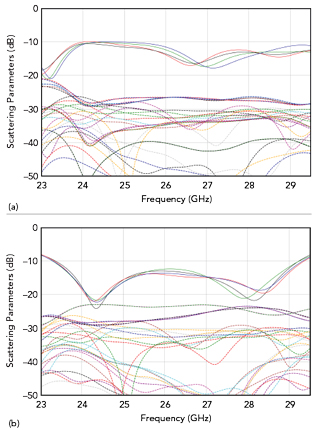
Figure 6 (a) Vertically polarized SIW antenna performance showing matching (solid lines) and isolation (dashed lines) at the IC ports. Horizontally polarized air waveguide antenna showing matching (solid lines) and isolation (dashed lines) at the IC ports (b).
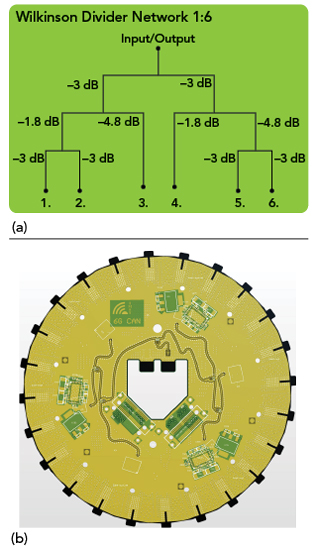
Figure 7 (a) Wilkinson divider network with distribution function. (b) Top-side antenna PCB including routed SIW network for vertical polarization.
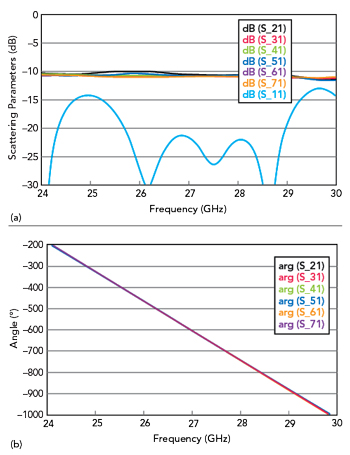
Figure 8 (a) Simulated Wilkinson divider network scattering parameter magnitude for vertical polarization (SIW). (b) Simulated Wilkinson divider network scattering parameter phase angle for vertical polarization (SIW).
Distribution Network
The beamforming IC allows the phase and amplitude of each radiator to be manipulated with 6-bit resolution. This results in a resolution of 5.625 degrees for the phase value and 32 steps for the amplitude. The signals of all the beamforming ICs on one PCB are summed with a Wilkinson divider network. To keep the coupling low, the signal for the vertical polarization is routed on the top layer, while the signals for the horizontal polarization are routed on the bottom layer of the PCB. Both signals are routed on microstrip lines. Since the six beamforming signals cannot be equally routed in a Wilkinson divider network, an asymmetric Wilkinson divider is used to achieve equal amplitude distribution of all feeding signals. Figure 7 (a) shows a sketch of the Wilkinson divider network and its distribution function. Figure 7 (b) shows the network routing of a vertically polarized SIW signal on the PCB. Figure 8 (a) shows the simulated results for the magnitude of the scattering parameters for the Wilkinson divider. Figure 8 (b) shows the simulated phase values for the Wilkinson divider. All the sum networks have similar routings, making the line lengths approximately the same to avoid amplitude or phase variation over frequency. The phase values for each path show a maximum deviation of ± 2.5 degrees and the amplitude variation is about ± 0.4 dB. The input reflection coefficient is below -13 dB over the entire operating frequency range.
On the input/output of the combing network, the microstrip signals are routed on a SIW transmission line to a backplane PCB where they are transformed to a stripline mode. The stripline path for each polarization is routed in different layers on the backplane PCB. The backplane PCB uses the same eight-layer stack-up as the antenna PCBs. A Wilkinson divider network is used to sum the signals on the backplane PCB. A four-to-one combining network designed for equal distribution can be used in this case. The combined signals are routed to the short edge of the backplane PCB. The stripline transmission lines on the backplane PCB are routed to a 2.92 mm coaxial connector that is used to feed the 5G CAN module.
The complete RF design of the module was done with the 3D EM simulation software EMPIRE-XPU.2 All the individual parts (antenna, PCB divider networks, RF transitions, backplane PCB, etc.) were designed independently as a first step. Next, integrations of these parts were simulated. As an example, the Anokiwave beamforming chip was simulated including eight corresponding antenna elements along with the antenna feed network. Finally, the complete module, including all PCBs with components and antennas was simulated to ensure that the module met the required RF performance. The complete module simulation was completed in a few hours thanks to the efficient simulation technique and simulation software.
SIMULATION RESULTS
The module achieves excellent performance for the whole frequency range from 24.25 to 29.5 GHz. The omnidirectional coverage in azimuth is achieved with 96 antenna beams, 48 beams for vertical and 48 beams for horizontal polarization. Each beam is created by a 4 × 6 antenna element tile that uses four PCBs and six antenna elements per PCB. The resulting 3 dB beamwidth is about 8.5 degrees in azimuth. Figure 9 (a) shows the simulated radiation characteristic for the horizontally polarized array (air waveguide) and Figure 9 (b) shows the same results for the vertically polarized array (SIW) at 27 GHz. The directivity achieved is about 19 dBi which results in an EIRP of about 50 dBm at P1dB.
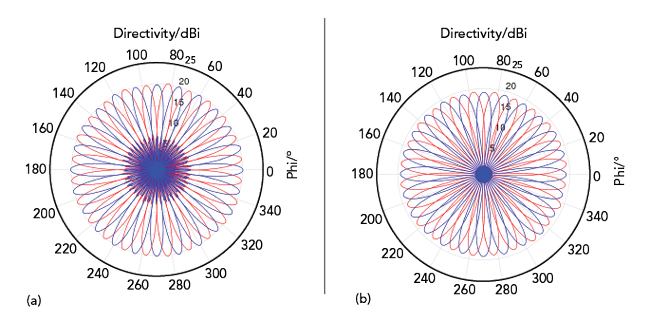
Figure 9 (a) Azimuth scan performance for horizontal polarization at 27 GHz. (b) Azimuth scan performance for vertical polarization at 27 GHz.
The scanning performance in elevation is about ± 30 degrees with less than 3 dB scan loss. Figure 10 shows the simulated 3D radiation characteristic for one beam that is scanned in the vertical direction.
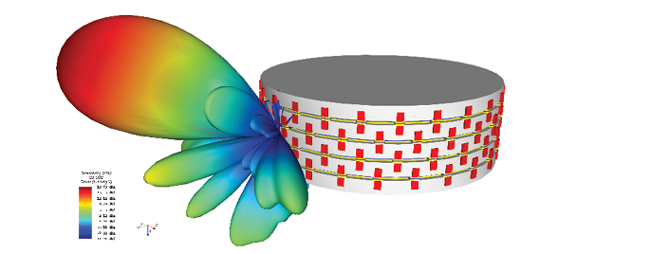
Figure 10 The 3D radiation pattern for an elevation scan for vertical polarization at 28 GHz.
Up to four simultaneous beams are supported, each set up from a quarter of the circular module. Figure 11 shows the simulated 3D radiation characteristic for two simultaneous vertically polarized beams at 26 GHz.
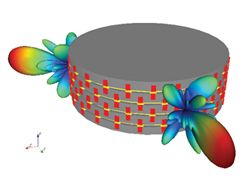
Figure 11 3D radiation characteristics with two simultaneous antenna beams.
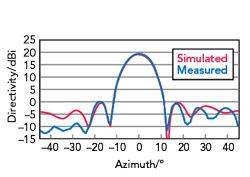
Figure 12 Simulated versus measured directivity for a vertically polarized beam in the azimuth plane.
MEASURED RESULTS
The module described in this article is intended for use as a development platform for 5G and 6G systems. It is available as an evaluation kit for 5G and 6G research teams, along with customers who are interested in other applications. Figure 12 shows the simulated versus measured directivity results for a vertically polarized beam in the azimuth plane. The result shows excellent agreement for scan angles less than ± 20 degrees. The deviation at larger scan angles appears to be related to calibration. To minimize this issue, IMST is still working on the best calibration method for the 5G module and the expectation is that the agreement with the simulation will improve as the calibration method is refined. More data will be available as the calibration method evolves.
CONCLUSION
The 5G CAN front-end module is a compact, flexible and scalable phased array front-end that can be used in different 5G and 6G scenarios. The module described operates over a frequency range from 24.25 to 29.5 GHz, covering the n258, n257 and n261 3GPP bands. The module provides omnidirectional coverage in azimuth and ± 30 degrees scanning in elevation. The design can be easily scaled in diameter to increase the EIRP as well as the number of beams. Upscaling the frequency to 39 GHz to cover 5G mmWave band n260 is also possible.
References
- W. Simon, D. Schäfer, S. Bruni, M.A. Campo, O. Litschke, “Highly Integrated Ka-Band Front-end Module for SATCOM and 5G,” 2019 IEEE APMC.
- A. Wien, “EMPIRE XPU Reference Manual,” assessed June 2023, Web: https://empire.de/resources/manual/.