The U.S. Department of Defense’s (DOD’s) Space Development Agency (SDA) is leading the rapid evolution of military space-based communications and sensing in response to the increasing threat from adversaries. Reliant on a small number of geosynchronous orbit (GEO) communication satellites that are now seen as “large, big, fat, juicy targets,” according to Gen. John Hyten, USAF, (Retired), the DOD is building a more robust and defendable solution known as the Proliferated Warfighter Space Architecture (PWSA). Comprised of hundreds of satellites, the PWSA provides expanded capabilities in a more resilient global architecture for communications, tracking and early warning capabilities. To respond to the urgent needs of today’s warfighter, SDA is pursuing aggressive timelines with a modest budget by embracing commercial trends like smaller satellites, shorter mission durations and the use of radiation-tolerant commercial components. York Space, Mynaric and CesiumAstro are a few of the non-traditional entities utilizing mass-manufacturing approaches to deliver space capabilities at commercial scale and they have partnered with SDA to deliver technology such as satellite buses, optical inter-satellite links and active electronically steered array (AESA) antennas. With a 2022 budget of $1.4 billion, SDA has gained the traction required to deliver new defense capabilities at the pace, budget and scale required to defend U.S. national interests and support the warfighter.
Enabling Capability in Layers
Architected in functional layers, the PWSA’s capabilities are enabled by a mesh network of communications satellites, sensors and data processing. The three primary layers utilizing RF and microwave technologies are the Transport, Tracking and Custody Layers, each of which includes dedicated satellites. Other layers may be hosted on existing satellites or combined with other payloads.
Transport Layer: Serves as the secure communication layer routing data across the network and to the warfighter. Envisioned to consist of 300 to 500 satellites in a high low earth orbit (LEO) ranging from 750 to 1200 km in altitude. High LEO provides a broader view of the earth and satellites in a lower orbit but it presents a more challenging radiation environment. User services will include both Link 16 and the Integrated Broadcast System, providing communications, threat warning and situational awareness.
Tracking Layer: Provides active sensing capabilities enabling real-time warning and battlefield awareness that includes 24/7/365 missile warning, missile tracking and missile defense (MW/MT/MD) capability. The orbits vary, depending on the mission. Data is relayed through the Transport Layer for aggregation and communication to the ground and user segments.
Custody Layer: Includes sensors that aggregate collected data over time, building strategic insights for left-of-launch (before launch) tracking and targeting. Data fusion from many sensors is networked through the Transport Layer to provide real-time insights to the warfighter.
Together, the layers form a cohesive network to provide a clear theater picture to the warfighter. Each layer contains a compatible set of core communications payloads that ensure connectivity to the Transport Layer, including both RF and free-space optical laser communications. Figure 1 shows a diagram of the PWSA architecture and its functional layers. The Transport Layer, Tracking Layer and Custody Layer are the most discrete layers utilizing RF and microwave technologies. Note that not all layers will have dedicated satellites and those functions may be shared across the constellation.
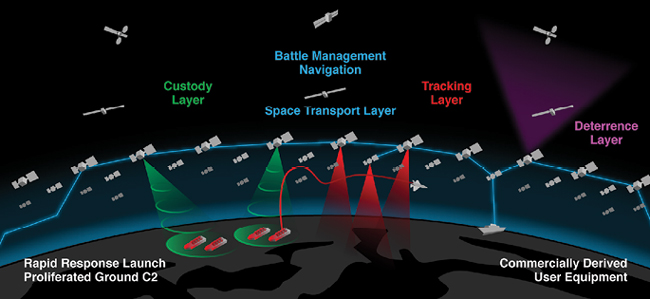
Figure 1 The PWSA architecture and its functional layers.
Semper Citius: Move Fast and Launch
Averaging 2.5 years from award to launch, SDA’s first batch of satellites is already in orbit. SDA is breaking the cycle of increasing cost and schedule typical of defense infrastructure. Taking a spiral development approach, SDA is deploying the PWSA in stages called tranches, with increasing technical capabilities and quantities of satellites to be delivered in each tranche. Figure 2 shows the timeline and current expectations of this tranche development approach.
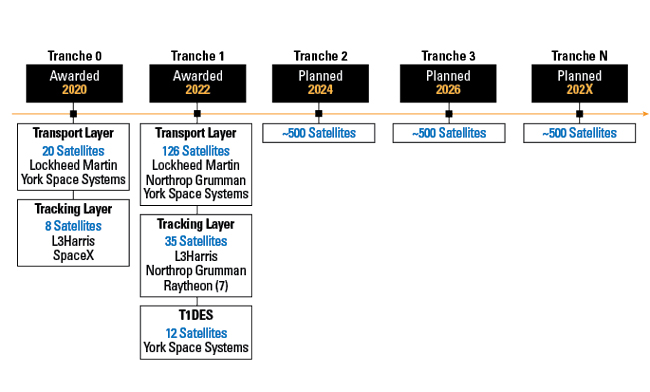
Figure 2 SDA spiral-based approach to constellation development.
Leveraging an agile approach reduces risk while providing a steady flow of opportunities to deploy new capabilities. Strategically focused on proving that the timeline and budgets are achievable, the first 10 Tranche 0 satellites launched on April 2, 2023. Tranche 1 awards were recently completed and are scheduled to launch in 2025, delivering enhanced communications and sensing capabilities enabling initial warfighting capability in specific regions of the globe. The first Tranche 2 satellites launch in 2027, providing full global capability, which will be followed every two years by Tranche 3 onward.
Recognizing the need to evaluate new technologies outside of the core deployment, SDA has established multiple experimental missions between tranches to support the development and evaluation of new approaches and technologies that, once matured, can fold into future tranches at scale. The focus of these technologies in the coming tranches will be alternative pointing, navigation and timing, improvements in target tracking and satellite meshing and advanced waveforms for communication.
As it slices through red tape while holding the line on the budget, the SDA acquisitions process has reduced typical acquisition times from years to months. Requirements are rapidly developed and drafts are published for feedback. Request for proposal (RFP) responses, historically due several months after issue, are due in as few as four weeks from publication. Proposals are evaluated and awards are granted at a similar pace, allowing awarded companies to hit the ground running. Earning a reputation for setting a schedule and then keeping it, companies bidding on PWSA RFPs are adapting their bid processes and planning cycles to provide more feedback during the draft phase. To compete, companies must engage their supply chains, build execution teams and rapidly draft responses tailored to the aggressive schedule required by PWSA deployment.
“One thing I can say for certain is that with regards to ideas, the more the better. And if you can look across the broad space ecosystem and pull in as many ideas as possible, regardless of the size of the vendor that’s promoting that idea, you’re going to be more successful at it putting solid innovative ideas on the plate,” said Gen. B. Chance Saltzman, U.S. Space Force, Chief of Space Operation.
Inviting Companies of All Sizes to the Table
To broaden input across the space ecosystem, SDA encourages the participation of non-traditional commercial companies in addition to traditional defense organizations. The goal is to pull in as many new ideas as possible and elevate the best contributions, regardless of the source. To level the playing field, SDA has committed to a multiple-solicitation, multiple-vendor approach to maximize participation, increase the number of meaningful awards and prevent vendor lock. Encouraging competition is intended to enhance supplier diversity, control costs, spur innovation and keep incumbents on their toes. The diversity of awards is impressive and benefits smaller or non-traditional companies, as they can participate and compete both with and against major defense primes with innovative new offerings. With each award announced, SDA provides the entire space community with a window into the schedule and price to win, an intentional move reinforcing its commitment to diversity and broad-based participation.
Communications Payload Technology Evolution
RF and microwave technologies are core to the PWSA by enabling mission communications, tactical communications and sensing capabilities. Figure 3 shows an architecture for a Tracking Layer satellite payload. This architecture shows several opportunities for digital, RF and optical components and systems.
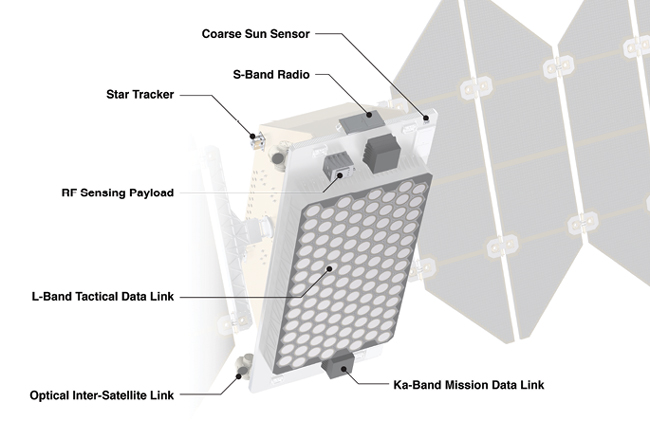
Figure 3 Example of a Transport Layer satellite configuration with common payload components.
A payload like that shown in Figure 3 may contain the following systems:
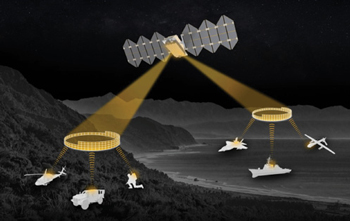
Figure 4 Satellite-enabled Link 16 supporting multiple battlespaces. Source: CesiumAstro.
Mission data link: enabled over military Ka-Band radio frequencies that provide focused, high data rate communications. Serving as the primary gateway connection, the mission data link utilizes gimbaled reflectors in early tranches. This limits connectivity to a single beam and it requires a high degree of pointing accuracy on the spacecraft.
Tactical data link: enables direct connectivity to the warfighter using the Link 16 multi-user communications standard on L-Band radio frequencies. To date, Link 16 has been restricted to ground, ship and airborne assets, but SDA is expanding its capabilities by adding payloads compatible with widely-deployed systems. Early tranches baselined fixed antennas requiring body pointing or gimbaled direct-radiating antennas. These capabilities are critical in advancing the first space-deployed Link 16 assets, but modernization through multi-beam phased arrays is needed to better support the needs of the warfighter with enhanced coverage areas, jamming resistance and reduced latencies, among other benefits. A rendering of a satellite-enabled Link 16 tactical data link is shown in Figure 4.
RF sensing: a key technology that is utilized in a wide range of missions and requirements. A deeper discussion on sensing for the SDA mission is available through the agency’s program solicitations.
Early tranches of the PWSA have prioritized schedule and cost to validate the entire end-to-end constellation concept. Future tranches will require increased capability. Implementing these new requirements and capabilities will be driven by experimental missions as the primary on-ramp for new technologies.
Enhanced Payload Development
CesiumAstro is currently working to provide SDA with multi-beam AESA capabilities for the Ka-Band mission data link and the L-Band tactical data link. Its Vireo AESA payload will be supplied for integration into seven Raytheon space vehicles as part of Tranche 1’s Tracking Layer. Building upon prior efforts, its L-Band Link 16–compatible AESA supports a modular approach utilizing a scalable tactical datalink solution connecting disparate networks. Optimized for space, the communication payload provides fast electronic steering with multiple beams, integration with commoditized buses and it meets associated requirements for size, weight, power consumption and thermal management. It communicates with fielded tactical terminals that were not designed to communicate with LEO. The system is being developed to accommodate large-quantity production in support of the constellation deployment goals while meeting the associated cost and schedule requirements. CesiumAstro’s design and production methods directly address the challenges associated with fielding the PWSA. The design leverages industry-wide investment in mobile communication for the cost and performance of ICs, meets requirements for high volume manufacturing and uses software-defined features.
While phased array antennas are being used in terrestrial applications, space introduces a more rigid set of design requirements, beginning with the need for greater power efficiency. Generating power in space is challenging in itself while dissipating the heat produced by the devices that use that power can be even more difficult. Thoughtful system engineering is required to optimize power generation and consumption, manage performance over wide temperature swings, maximize RF performance and minimize overall mass. For example, while current SOI, GaN and GaAs processes are naturally radiation-tolerant, their RF performance can vary widely with temperature. These challenges must be addressed with a highly integrated interdisciplinary engineering team that includes systems, RF, electronics, digital, software, thermal, packaging, manufacturing and test engineers that collaborate on a tightly integrated, yet modular design that meets mission requirements and is manufacturable at scale.
AESA Communications Revolution
The use of AESA technology for communications has multiple benefits for the warfighter and the mission. Early tranches favored fixed antennas that required body pointing or gimbaling to provide single beams of connectivity. The use of multi-beam AESAs, once an expensive radar solution, is now a mature communications technology. AESA payload architectures offer several operational benefits:
Multiple simultaneous beams: Maximize capacity and frequency reuse with multiple independent beams from a single aperture.
Fast steering: Rapidly hop and steer beams to improve spectrum utilization and increase user capacity.
Beam shaping: Full amplitude and phase control at each element allows for shaped beams, which optimize coverage area and reduce sidelobes or create nulls for interferers.
Arbitrary polarization: Fully polarimetric elements can be used for isolation between channels, to correct polarization over scan angle or minimize polarization mismatch for ground terminals.
Graceful degradation: Redundancy minimizes the impact of single-component failure on overall performance, extending mission life.
In addition to these direct benefits for communications, AESAs simplify the overall spacecraft architecture by reducing the complexity of the attitude determination and control system (ADCS). In a study of small satellite failures from 2000 to 2016, ADCSs were the second-leading cause of partial or complete mission failures. A gimbaled or body-pointed antenna imposes more stringent requirements on an ADCS, increases the cost and complexity of that system, increases the power requirements and reduces the average vehicle lifetime. Utilizing an AESA reduces the size, cost and complexity of the ADCS while providing pointing redundancy in the event of reduced ADCS performance, which can happen when the spacecraft is in “safe hold” mode. AESAs also allow a spacecraft to implement much simpler and more straightforward pointing control strategies and perform other functions while executing communications tasks. For example, a spacecraft can simply nadir-point, a process of pointing in the downward field of view of that satellite, while tracking one or more ground terminals. This process can result in a significant reduction in programming and operational overhead. Furthermore, a spacecraft can more easily track the sun while communicating with multiple users, simultaneously maximizing quality of service, power generation and energy storage. Because of these benefits, AESA antennas are now being considered for Link 16 communications and other payloads as an approach to optimizing total system lifecycle cost and performance.
Conclusion
SDA’s vision has been laid, the path from solicitation to launch has been proven and policymakers have aligned the budget to support its goals. DOD acquisitions reform is taking hold and new capabilities are moving from concept to flight at a record pace. Companies of all sizes are contributing new, innovative capabilities to support the warfighter and defend the nation. SDA’s layered function model and tranche-based deployments provide a continuous flow of opportunities to design, evaluate and deploy new capabilities on orbit. Now is the time for the RF and microwave community to deliver the innovative communications systems and sensors needed to enable this ambitious mission set. RF and microwave electronics and antenna technologies are core to the mission of providing enhanced communication capability and situational awareness at scale to today’s warfighter. It is increasingly clear that space is vital to our way of life and national security. Maintaining and extending our advantage in space is a national imperative and critical to paving the long-term path to peace and prosperity.