
Frequency converters are rapidly becoming the only analog building block in a variety of RF and microwave systems. This trend is obvious in wireless consumer products, instrumentation, radar and radar-warning systems, telemetry and secure communications. The advent of synthetic instruments puts frequency converters at center stage within the RF architecture of test systems. A synthetic instrument is a concatenation of hardware and software modules used in combination to emulate a traditional piece of electronic instrumentation, such as a spectrum analyzer or a signal generator. This definition is derived from meeting notes of the Synthetic Instruments Working Group, a joint participation between the Department of Defense, Defense Prime Contractors and Suppliers. Performance parameters relating to frequency converters are quite numerous and can only be fully optimized with complex, multi-stage architectures. The purpose of this article is to provide understanding for the trade-offs in performance involved in using simpler, less costly architectural approaches. This will help select and devise frequency conversion solutions that are optimized for specific classes of applications. For example, a downconverter aimed at surveillance of the airwaves and identifying specific signals in the presence of a multitude of others has different requirements from a test and measurement application where signals are few and known. The introduction of high frequency quadrature demodulators, such as the LTC 5515, direct quadrature demodulator from Linear Technology, raises the opportunity to simplify downconverter block diagrams. Similarly, quadrature modulators, such as the HMC495LP3, direct quadrature modulator from Hittite Microwave make it equally possible to reduce the complexity of upconverters. This article is intended to shed light on some of the key performance parameters and to help system designers and integrators identify appropriately priced solutions to meet their needs. Some performance parameters related to frequency converters are directly tied to the complexity of the block diagram. It will also be seen that a great many of the most significant parameters are independent of the approach taken. It is therefore possible to obtain low phase noise, accurate conversion gain and good modulation quality from a single-stage, lower cost converter.
Categorizing Converter Transmission and Signal Quality Parameters

Table 1 shows parameters that define the transmission quality of a converter categorized in a logical manner. How only spurious signals and responses are affected by the architecture will be discussed. All other parameters can be just as easily optimized in a simple architecture. One could even argue that a configuration with shorter and fewer signal paths is better suited for optimizing transmission parameters such as amplitude and phase response.
Performance Factors Dictated by the Architecture
The following parameters are definitely favored in a multi-stage design:
-
Spurious responses (downconverters)
-
Spurious sidebands (upconverters)
-
Frequency range
-
Port isolation

Performance Factors that Can Be Enhanced by Calibration and Signal Processing
-
Conversion gain accuracy
-
Conversion gain uniformity with respect to input or output frequency
-
Signal level accuracy
-
Linear modulation parameters such as amplitude and phase response over the bandwidth occupied by the signal
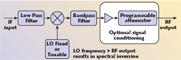
Performance Factors that Can Be Enhanced by Technology Regardless of Architecture
-
Noise floor, through the use of low noise amplifiers
-
Enhanced image frequency suppression, through the use of YIG-tuned input filters
-
Phase noise

Single-stage Block Converter Design Example

A block converter takes a frequency band and converts it to another one and preserves the extent of the band. For example, an input band ranging from 4.9 to 6.0 GHz may be downconverted to 1.1 to 2.2 GHz, as shown in Figure 1, or the reverse upconversion process can take place, as depicted in Figure 2. In the case of the block downconverter, one relies heavily on the selectivity of the low pass filter for isolating the LO from the input port as well as suppressing the image frequency. In the case of the upconverter, the low pass filter (LPF) at the input is more straightforward, since the LO frequency is farther removed from the IF input. The bandpass filter, on the other hand, ensures isolation between the LO and the output RF port as well as the selection of the desired lower sideband.
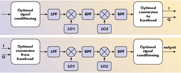
Single-stage Tunable Converter
Consider the configurations in Figures 3 and 4, which represent a straightforward single-stage up or downconverter. The selection of IF and LO frequencies has a direct influence on the spurious response (downconverter) and spurious sidebands (upconverter). The higher the IF frequency, the easier it is to filter unwanted sidebands (upconverter) and prevent signals at LO + IF from getting into the mixer. The latter is known as image frequency suppression and becomes practically impossible when the IF frequency is too low. Also shown are optional stages of IQ (or quadrature) demodulation and modulation for converting directly from baseband to IF, if needed. Direct conversion is used extensively in consumer products because it is economical in parts count, size and power consumption. In this case, it offers the added benefit of coping with the spectral inversion issue. This is done by interchanging the I and Q signal ports.

Two-stage Design Example
The multi-stage architectures (see Figures 5 and 6) offer some benefits:
-
Easier filtering to protect against spurious responses in downconverters.
-
Easier filtering to suppress unwanted sidebands, such as LO feed through, alternate sideband and other undesired out of band signals.
-
Possibility of covering a frequency range with LOs having a more limited range than in the simpler architecture.
-
Better isolation between input, output and LO ports.
-
When each of the two stages undergoes a spectral inversion, the resulting spectrum at the output is unchanged.
Spectral Inversion

Spectral inversion in frequency conversion occurs whenever an increase in the frequency of the input signal results in a decrease of the frequency of the output signal. This is usually the case when the output frequency is below that of the LO. The effect of spectral inversion is seen in Figures 7 and 8. When the I and Q baseband signals are used on the input for an upconverter or on the output for a downconverter, the spectrum can be redressed simply by interchanging the I and Q ports. It is possible to implement the equivalent of the interchange of I and Q in software when processing the signal.
Impact of LO on Architecture
This topic requires much more elaboration than the scope of this article allows. Very often, the block diagram configuration is dictated by LO considerations. In particular, one generally tries to use the narrowest possible frequency range for the LO to limit design complexity, cost, size and power consumption.
The narrower range of the LO is circumvented in one of two ways:
-
Multiply the LO output to extend the frequency range. This leads to complications in filtering subharmonic sidebands sufficiently to reduce unwanted effects such as spurious sidebands (upconverter) and spurious responses (downconverter).
-
Add switched IF paths to extend the frequency range while avoiding the undesired effects mentioned above.
In general, the LO(s) are the main contributors to phase noise and are therefore selected or designed to produce desired levels of phase noise. The trade-offs with respect to block diagram complexity can be quite significant since the phase noise fares better when the frequency range is limited. The LO is a key component in the design of converters and generally drives cost, size and time to market.
Comparison Between Architecturally Determined Performance Parameters for Single- and Multi-stage Frequency Converters

It is clear from Table 2 that the multi-stage architecture is superior in protecting the output port from signals present on the input port or the LO. All other parameters are independent of the architecture. This is quite significant, since it opens up the possibility of using less expensive units with a smaller footprint and lower power consumption in a variety of applications. One such application is dedicated test systems. In this instance, the number of signals present is limited and known in the great majority of cases. For example, the presence of an LO component far removed from the signal of interest is of little consequence, so long as it does not introduce intermodulation products or interfere with the accuracy of the measurement. It should be noted that everyone is familiar with the signal spike on the far left of the screen of a spectrum analyzer caused by LO leakage into the IF. It has become an indicator of zero frequency. A counter example is a radar system destined to work in hostile environments. Susceptibility to signals on the input leaking to the output raises the possibility of jamming signals rendering the radar ineffective. A multi-stage approach with appropriate protection is better suited for this application. It is important to note that a converter required to cover a frequency range of several octaves is unlikely to be realized effectively by means of a single-stage, single IF path architecture.
Conclusion
For applications that tolerate some level of spurious responses (downconverters) or out-of-band spurious sidebands (upconverters), a simple one-stage converter solution may be all that is required. The consequences in time to market, cost, size and power consumption may be very significant.
Roland Hassun is an independent technical consultant specializing in test and measurement, frequency synthesizers and converters. Prior to consulting, he worked for HP/Agilent, where he was involved in pioneering several areas of instrumentation, including low noise RF signal generators, high speed digital waveform generation and direct digital synthesis.