The remaining phenomenon to hinder radio link performance with highly directive beams is the thermal deformation of the tower mast holding the antenna. Daily sun heating occurs predominantly with clear skies. Standard monopoles bend from 0.3 to 0.6 degrees because of the temperature difference between the side of the monopole heated by the sun and the opposite side. Structures other than monopoles, such as lattice towers and buildings, are usually less prone to this effect; however, requiring that two- and three-foot E-Band antennas be mounted to specific rigid structures is not an acceptable constraint.
EMBS ANTENNA
Since the daily thermal change is slow and limited in angle, an electromechanical beam steering (EMBS) antenna can compensate for the thermal behavior. Using a simplified model for a parabolic dish without a sub-reflector,4 the limited angular tilt, θ, of the beam from boresight is introduced by offsetting the focus, δ, on its plane:
Scan loss in the far field translates to a gain loss as a function of steering angle; hence, scan loss is the figure determining the practical applicability of a steering antenna. Simulations characterizing the scan loss show the EMBS antenna design has under 3 dB scan loss at 2 degrees, which is acceptable since monopoles are expected to deform less than ±1 degree.
Using actuators to offset and rotate the sub-reflector in a Cassegrain antenna is equivalent to a steering mechanism and easier to implement, so it was chosen for the EMBS design. In a basic yet effective form, the tracking algorithm in the antenna control system monitors the received power signal and drives the actuators, altering the sub-reflector configuration to maintain correct alignment. The received signal strength indication signal is used since it is available at the outdoor unit (ODU). With most commercial E-Band radio systems, no other connections are required, which keeps the antenna and ODU as separate entities.
TESTING THE CONCEPT
To validate the EMBS concept, an open test range was set up using a 210 m link between SIAE Microelettronica’s laboratory rooftop and a tower site with a monopole structure. A standard one-foot antenna was installed on the tower, and a three-foot EMBS prototype was installed on the rooftop (see Figure 3). The E-Band prototype antenna included an actuator and control system to change its alignment with the antenna on the tower.

Figure 3 Test range comprising a typical 30 m high monopole for telecom backhauling (a) and three-foot EMBS prototype antenna (b).

Figure 4 Power loss caused by antenna misalignment (a); improvement achieved with EMBS (b).

Figure 5 Simulated instantaneous uptime over 24 hours for the standard three-foot antenna.

Figure 6 Simulated instantaneous uptime over 24 hours for a 3.5 km link using three-foot antennas equipped with EMBS.
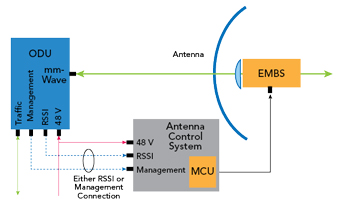
Figure 7 Connecting the EMBS antenna (antenna control system, actuators and antenna) to the ODU.
Initially, the EMBS antenna was set up as a standard three-foot antenna, with the actuator locked to its nominal position, making the beam coincident with the antenna’s axis. The antenna orientation was set by adjusting the antenna’s coarse and fine alignment screws, noting the received power as the reference power level. Once peak alignment was achieved, the three-foot antenna was misaligned by about 0.63 degrees, leading to a significant power loss (see Figure 4). The measurements agree with theory, which projects approximately 20 dB loss from the aligned reference value. The manual misalignment was repeated in all four directions to mimic severe monopole thermal deformation with a standard three-foot antenna. Next, the EMBS antenna actuators were enabled and used to maximize the received power after the same manual misalignment in the four directions. The relative power loss was measured and is also shown in Figure 4.
The measured loss levels with the active EMBS antenna show that the EMBS solution provides an effective way to counter monopole deformation, which would otherwise degrade link performance.
To show the effectiveness of the EMBS antenna in the field, SIAE Microelettronica developed a model for thermal deformation to simulate a 3.5 km link using three-foot antennas. The link was planned using standard point-to-point margins and alignment errors, designed to meet 99.995 percent availability operating at 400 Mbps throughput (4-QAM using a 2 GHz channel with reduced bandwidth) and 99.95 percent availability at 10 Gbps throughput (128-QAM using a 2 GHz channel bandwidth). The worst case scenario for the two sites occurs when the structures tilt in opposite directions, resulting in the largest loss. Figure 5 shows the simulated performance over 24 hours for a link using standard antennas. Incorporating minor seasonal variations to extending this over a full year, the expected link availability drops to 99.990 percent at 400 Mbps and 96 percent at 10 Gbps. This means 35 hours per year of lower capacity, which is unacceptable for mobile applications. The 22 dB loss is greater than the 9 + 9 dB added gain from using three-foot antennas instead of one-foot antennas.
With the same assumptions, Figure 6 shows the expected performance with the EMBS antenna compensating for deformations due to heating, including residual misalignment and realistic scan losses. Including a small 1 dB equivalent loss, the link’s uptime over a year meets the required 99.995 percent availability at 400 Mbps and the availability of a 10 Gbps link is 99.95 percent. An EMBS system with the performance demonstrated in this field experiment can overcome thermal deformations of monopoles, enabling the use of higher gain antennas for long-reach mmWave links.
CONCLUSION
Larger antenna sizes at 80 GHz can enable higher capacity and low latency point-to-point links for mobile and other wireless transport applications such as 5G. However, the standard high directivity antennas have drawbacks from misalignment and thermal deformations, which will severely limit their usage, as predicted by simulations and confirmed by measurements. An EMBS approach, as described in this article, counters thermal misalignment and enables use of high directivity antennas, i.e., 2 ft and greater. The prototype three-foot EMBS antenna achieved low static and scan loss, i.e., approximately 1 dB at 0.63 degrees off boresight. Tracking to counter misalignment can be driven by the radio equipment or the antenna controller (see Figure 7).
While the solution described here only uses information from the local site, more effective tracking might be achieved if the control algorithm coordinates information from both the local and remote sites to align both local and remote antennas. To coordinate the remote site, the ODU could send transmit power adjustment signals to counteract fading, preventing unnecessary antenna movement. Also, an ODU with MEMS devices could provide tilt direction indications for a faster reaction of the first movement.
References
- Nordrum et al., “5G Bytes: Millimeter Waves Explained,” IEEE Spectrum, May 2017, spectrum.ieee.org/video/telecom/wireless/5g-bytes-millimeter-waves-explained.
- Sharma et al., “Handbook of Reflector Antennas and Feed Systems,” Artech House, 2013, ISBN 160807515X.
- Visser et al., “Array and Phased Array Antenna Basics,” John Wiley & Sons, 2006, ISBN 0470871180.
- Lo et al., “Array and Phased Array Antenna Basics,” John Wiley & Sons, 2006, ISBN 0470871180.