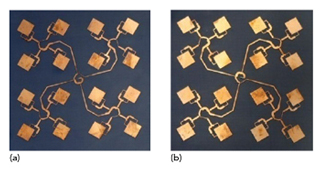
Figure 3 Prototype 4 × 4 arrays for LHCP (a) and RHCP (b).
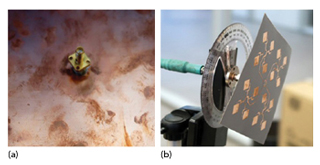
Figure 4 Antenna array backside (a) and mounted on a tripod with SMA rotary joint for measurements (b).
The results of electromagnetic simulations and measurements of antenna array gain and AR variation are shown in Figures 5 and 6, respectively. Array gain was calculated from the free-space path loss between two identical antennas. A maximum gain of 16.2 dBi was measured at 9.9 GHz, which agrees with the maximum simulated gain of 17.2 dBi. Minor discrepancies between the measured and simulated results are attributed to imperfect measurement conditions and manufacturing variations. Due to the narrowband nature of the patch radiating elements, the array gain decreases toward the lower and higher frequencies of the measurement range. The maximum gain at 9.6 and 10.1 GHz was around 13 dBi, and the radiation efficiency was between 69 and 75 percent over the same band. The measured cross-polarization isolation was better than 29 dB from 9.5 to 10.1 GHz, with the highest value of 41 dB at 9.9 GHz. The front-to-back ratio at the same frequency was around 28 dB.
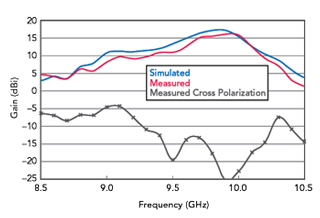
Figure 5 Measured vs. simulated gain and measured cross polarization.
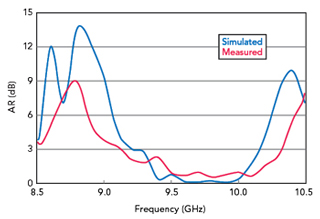
Figure 6 Measured vs. simulated axial ratio vs. frequency.
AR was determined by measuring the variations of the received signal while rotating either of the two antennas about its boresight axis. The variation under full rotation was within 1 dB across a 3 percent bandwidth (9.5 to 10.15 GHz), which corresponds to the range where gain variation was less than 3 dB. AR was less than 3 dB from 9.1 to 10.3 GHz, representing a 12.3 percent bandwidth. As the AR bandwidth of patch antennas is typically only a few percent, this is notably better than what is typically achieved with single-layer patch radiating elements. Because parasitic radiation degrades the purity of CP, the structure’s high degree of central symmetry preserves low ARs by enabling mutual compensation of parasitic radiation from different parts of the FN.
Figure 7 shows the measured and simulated |S11| of the antenna array, with -19 dB at the center frequency and better than -10 dB from 9.0 to 10.3 GHz. Both measured and simulation display excellent impedance match from 9.5 to 10 GHz, where |S11| ≈ -20 dB. Measured and simulated radiation patterns of the array in two orthogonal planes at 9.9 GHz (see Figure 8) display good agreement and excellent pattern symmetry.
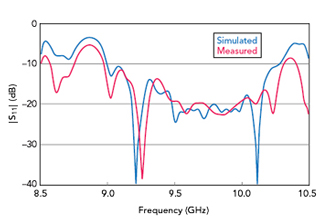
Figure 7 Measured vs. simulated |S11| vs. frequency.
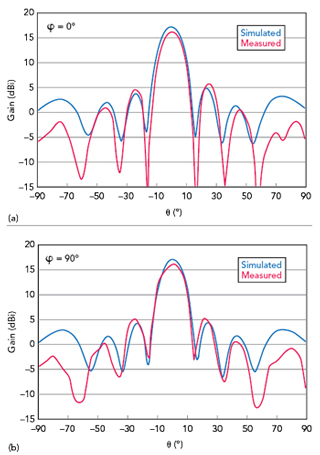
Figure 8 Array measured vs. simulated radiation patterns at 9.9 GHz, for φ = 0 (a) and 90 (b) degrees.
CONCLUSION
The CP printed 4 × 4 antenna array with a double sequential rotation FN has a high degree of central symmetry, achieving excellent return loss and good circular polarization over a broader range of frequencies than typically attainable without the use of multilayer patch configurations or stacked multi-resonator microstrip patch antennas. Due to the structure’s simplicity, fabrication uses conventional photolithographic techniques, making the design suitable for low-cost, reproducible mass production. In cases where additional gain is needed, the 16-element array can be used as a subarray in larger arrays with multilevel sequential rotation FNs. Intended for X-Band communication systems, the design approach can be applied to microstrip antenna arrays operating in other microwave frequency ranges.
ACKNOWLEDGMENT
This work was supported by the Serbian Ministry of Education and Science within the Technological Development Project TR 32024.
References
- R. Garg, P. Bhartia, I. Bahl and A. Ittipiboon, Microstrip Antenna Design Handbook, Second Ed., Artech House, 2001.
- H. W. Lai, D. Xue, H. Wong, K. K. So and X. Y. Zhang, “Broadband Circularly Polarized Patch Antenna Arrays with Multiple-Layers Structure,” IEEE Antennas and Wireless Propagation Letters, Vol. 16, July 2016, pp. 525–528.
- T. Yu, H. Li, X. Zhong, T. Yang and W. Zhu, “A Wide Bandwidth Circularly Polarized Microstrip Antenna Array Using Sequentially Rotated Feeding Technique,” Proceedings of the International Symposium on Antennas and Propagation, Vol. 2, October 2013, pp.743–745.
- U. R. Kraft, “An Experimental Study on 2×2 Sequential-Rotation Arrays with Circularly Polarized Microstrip Radiators,” IEEE Transactions on Antennas and Propagation, Vol. 45, No. 10, October 1997, pp.1459–1466.
- D. L. Nguyen, K. S. Paulson and N. G. Riley, “Reduced-Size Circularly Polarized Square Microstrip Antenna for 2.45 GHz RFID Applications,” IET Microwaves, Antennas & Propagation, Vol. 6, No. 1, January 2012, pp. 94–99.
- A. Chen, Y. Zhang, Z. Chen and C. Yang, “Development of a Ka-Band Wideband Circularly Polarized 64-Element Microstrip Antenna Array with Double Application of the Sequential Rotation Feeding Technique,” IEEE Antennas and Wireless Propagation Letters, Vol. 10, November 2011, pp. 1270–1273.
- S. Gao, Y. Qin and A. Sambell, “Low-Cost Broadband Circularly Polarized Printed Antennas and Array,” IEEE Antennas and Propagation Magazine, Vol. 49, No. 4, August 2007, pp. 57–64.
- M. S. Khan and F. A. Tahir, “A Circularly Polarized Stacked Patch Antenna Array for Tracking Applications in S-Band,” European Conference on Antennas and Propagation, April 2015.
- T. A. Milligan, Modern Antenna Design, Second Ed., John Wiley & Sons Inc., 2005, pp. 316-317.
- R. B. Waterhouse, Microstrip Patch Antennas: A Designer’s Guide, Springer Science+Business Media New York, Kluwer Academic Publishers, 2003, pp. 61–65.