MULTI-CATR SETUP
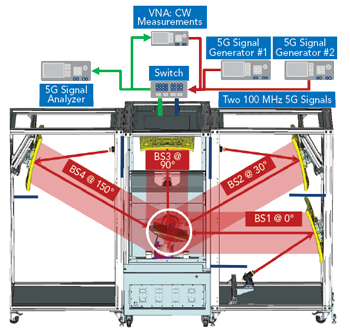
Figure 3 Multi-CATR system comprising four reflectors with components inside a shielded anechoic chamber.
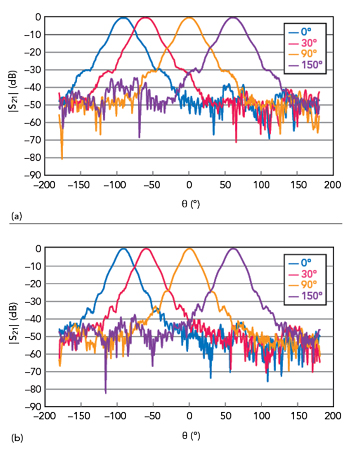
Figure 4 Vertical multi-CATR system antenna pattern measurements for the 20 dBi standard gain horn DUT at 28 (a) and 40 (b) GHz.
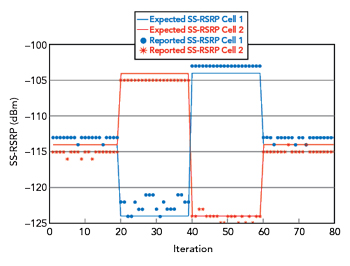
Figure 5 RRM test showing inter-frequency SS-RSRP reporting for two cells with different AoAs to the DUT.
A multi-CATR prototype with 3D positioning is designed, minimizing the system footprint while increasing the quiet zone size by 50 percent (see Figure 3). The reflectors and feed antennas are individually aligned using lasers at the positioner in the center of the quiet zone, which reduces measurement uncertainty from feed and reflector misalignment. The quiet zone uniformity of the multi-CATR system was assessed using two methods: the 3GPP method of quiet zone characterization and 2D field scanning of the amplitude and phase, used to extract the linear taper plus ripple.
The 3GPP method requires 322 separate 3D pattern measurements as a function of DUT angular and spatial placement, and the total radiated power variation is limited to 0.6 dB. The 3GPP quiet zone measurement uncertainty metric includes several factors, such as positioner influence, feed misalignment, chamber size, absorber thickness and the effects of additional objects placed in the chamber - such as a link antenna. The average taper and ripple across the FR2 band were 0.8 and 0.2 dB, respectively. The 3GPP quality of quiet zone measurement uncertainty measurements were performed using an A-Info LB180400-10 low gain horn antenna as the DUT, resulting in an average quality of 0.3 to 0.4 dB from 23.45 to 40.8 GHz and an average cross-polarization feed uncertainty of less than 0.07 dB, calculated according to 3GPP.7
As shown in Figure 3, in the vertical multi-CATR system, the four CATR setups were arranged inside a portable anechoic chamber at the specified probe angular locations along a vertical arc above the DUT, which was mounted on a 3D positioner. To prevent scattering from adjacent rolled edges into the quiet zone, absorber blockers were placed between all neighboring reflectors and below the reflector at 150 degrees. The system footprint is 3.25×1.4 m with a height of 2 m. The free-space path loss is 62.15 dB at 40 GHz, improving the dynamic range by almost 30 dB compared to the direct far-field approach for an equivalent quiet zone of 30 cm diameter, where the direct far-field has a range length of 24 m with a free-space path loss of 92 dB.
MEASUREMENTS
Four, dual-polarized, CATR feed antennas with eight cables were connected to a R&S OSP120 switching platform, with the cable outputs routed to the appropriate measurement instruments for the benchtop and vertical multi-CATR prototypes. This enabled the measurement instruments to connect to any single or pair of feed antenna polarizations, with a switching time under 10 ms. The system performed two sets of measurements:
Non-signaling with CW - A four-port R&S ZVA67 vector network analyzer measured the antenna gain patterns using a 20 dBi standard gain horn as the DUT. This measurement setup evaluated the similarity of the gain patterns among the reflectors at different frequencies.
Signaling with an active wireless device - A non-standalone (NSA) 5G wireless device was used to monitor the signal levels between different pairs of base stations at a single position. In NSA mode, the 5G wireless device uses an LTE base station for signaling and control of the device. The LTE link antenna in the prototype multi-CATR system was the R&S TS-F24V3 single-polarized Vivaldi antenna connected to the R&S CMW500 communication tester for LTE signaling. The CMW500 tester sends and receives the 5G FR2 base station signal to the 5G wireless device.
Figure 4 shows the antenna patterns measured with the non-signaling CW signals. For brevity, only the patterns for the H-Plane of the standard gain horn antenna at 28 and 40 GHz are shown. The similar patterns among the four reflectors at the two frequencies demonstrate the applicability of the proposed multi-CATR concept.
The last set of measurements evaluated the accuracy of the SS-RSRP measurements for a scenario of two active cells, using the multi-CATR system with a commercial wireless device supporting the 5G FR2 bands. The measurements were repeated 33 times for statistical significance, following the recommendations of 3GPP TS38.533 Annex G.5 (A discussion of other measurement scenarios was published previously and is not included here.3)
In the RRM test scenario with two active base station cells - two angles of arrival at the wireless device - the transmit power level was changed ±10 dB every 20 iterations to determine if the wireless device could accurately monitor both base stations simultaneously. The CATR setups at 0 and 90 degrees were used for this scenario, and the wireless device was placed so its rear portion faced 45 degrees, i.e., between the two CATR setups. The wireless device was able to simultaneously receive signals in multiple directions, even when one had significantly higher power than the other (see Figure 5). The measurements show both cells are reporting within 1 dB of the expected SS-RSRP for the higher transmit power and greater signal-to-noise ratio and within 1 to 3 dB of the expected SS-RSRP for the lower transmit power and lower signal-to-noise ratio. Although the gain for different orientations and polarizations of this commercial wireless device is unknown, the expected values assume the 3GPP requirements are met by the commercial product.
Figure 5 shows the 10 dB switch between transmit powers is correctly reported without delay. The lowest expected SS-RSRP level of -124 dBm is below the reference sensitivity limit defined by 3GPP, and the internal noise of the commercial wireless device results in higher measurement error for the lower transmit power. The reported SS-RSRP for cell 1 is in general higher than for cell 2, matching the results from the first RRM test scenario, where the SS-RSRP reports for the reflector at 30 degrees are also higher than for the reflector at 150 degrees. Reviewing these results, the commercial wireless device has higher antenna gain when the signal is received from the top of the device, which is consistent with the antenna module placement.
CONCLUSION
The proposed multi-CATR system performs accurate measurements for different types of DUTs operating in either passive non-signaling or active signaling modes and for both a single angle of arrival and multiple simultaneous angles of arrival. The proposed system has low quiet zone measurement error using multiple reflectors and types of measurement instruments and signals. By minimizing the disturbance from adjacent reflectors, combined RF and multiple angular measurements can be performed with a single measurement system. Consequently, a range of new applications for the multi-CATR system are feasible:
- With separate transceivers for each reflector, spatial MIMO measurements can be performed.
- With additional displaced feeds from the focal point to shift the plane wave direction by 5 to 10 degrees, RF fading scenarios can be constructed for 5G wireless device characterization.
- Intelligent reflecting surfaces (IRS), proposed for 6G, can be measured by assigning a subset of reflector(s) as base stations and the remaining subset as user devices, to evaluate the complete performance of the IRS for users and base stations located in the far-fields of each other.
- Placing antennas in other frequency bands at different reflector angles enables simultaneous wideband measurements from 6 to 140 GHz, which are important to measure spurious out-of-band emissions with in-band radiation performance.
Acknowledgments
The authors thank the following Rohde & Schwarz team members for assistance with conceptual discussions and measurements: Jose Fortes, Engelbert Tyroller, Anes Belkacem and Mert Celik.
References
- 3GPP, “Requirements for Support of Radio Resource Management,” ETSI, V16.0.0, 2019.
- 3GPP, “Verification of Radiated Multi-Antenna Reception Performance of User Equipment (UE),” ETSI, V14.5.0, 2017.
- C. Rowell, B. Derat and A. Cardalda-García, “Multiple CATR Reflector System for Multiple Angles of Arrival Measurements of 5G Millimeter Wave Devices,” IEEE Access, Vol. 8, 2020, pp. 211324–211334.
- “On IFF Method for Multi-AoA Test Setup for RRM,” Rohde & Schwarz, 2019.
- 3GPP, “UE Conformance Specification; Radio Resource Management (RRM),” ETSI, V16.1.0, 2019.
- W. Burnside, M. Gilreath, B. Kent and G. Clerici, ‘‘Curved Edge Modification of Compact Range Reflector,’’ IEEE Trans. Antennas Propag., Vol. AP-35, No. 2, February 1987, pp. 176–182.
- 3GPP, “User Equipment (UE) Conformance Specification; Radio Transmission and Reception; Part 2: Range 2 Standalone,” ETSI, V16.5.0, October 2020.