A foldable, deployable 5G sub-6 GHz (3.3 to 7.0 GHz) switched-beam “smart” base station antenna uses dual parabolic cylindrical reflectors without any active components. The azimuth plane is divided into three or four 120 or 90 degree main sectors to form a switched-beam smart base station antenna covering 360 degrees. Each unit can generate three (triple), four (quad), five (penta) or more beams (subsectors) in each 120 or 90 degree main sector. Each unit can simultaneously provide vertical and horizontal sectorization. A 3D printed 80 cm x 37 cm x 20 cm prototype with reflecting portions or faces covered by a very thin layer of aluminum foil tape generates from nine to 60 beams to cover the entire azimuth plane with 18 to 120 ports (±45 degree polarization), distributed in one, two or three vertical sectors, based on requirements. The peaks of the upper beams can be adjusted to be above the nulls of the lower beams and vice versa, so every user will always be close to the peak of a beam. Remote electrical tilt (RET) with arbitrary vertical and horizontal tilt angles can be separately applied to each beam. The number of generated beams, their configurations and the tilt angle of each can be changed by modifying the number of feeds and their locations without replacing the antenna. The antenna is gridded, which significantly reduces wind loading and lowers the weight of each multi-beam unit to 2 kg. The overall weight of the antenna, consisting of three or four units is about 6 or 8 kg.
A switched-beam smart antenna creates narrow beams, limited to a fixed number of scan directions to which the system switches depending upon mobile user location. The multi-beam passive method can be thought of as splitting the standard 120 or 90 degree main sector into narrower horizontal and, probably, vertical subsectors. This means that network planners must determine how many subsectors are required, with one beam for each. Different techniques may be used.1-4
Currently, switched-beam smart base station antennas use several frequency dependent components and, therefore, have low frequency bandwidth. In some systems, very complicated techniques have been developed using multi-beamforming networks, such as Butler matrices, to drive planar arrays of radiating elements.1,2 Luneburg lenses have also been used for beamforming.3,4 Luneburg lenses are composed of layered structures of dielectric concentric shells, each with a different refractive index. The cost of a classic Luneburg lens is high, and the production process is extremely complicated.
In this work, a foldable, deployable sub-6 GHz (3.3 to 7.0 GHz) 5G switched-beam base station antenna is described. It consists of two parabolic cylindrical reflectors and a set of small broadband resonant feeds.5 GTD® software code is written for dual parabolic cylindrical reflectors and verified experimentally for different configurations and applications.6
Multi-beam technology can be easily applied to the dual parabolic cylindrical reflector antenna by adding multiple feeds (see Figure 1). Shifting the location of any feed away from the focus of the subreflector results in tilting the beam generated by that feed. Hence, each beam can be easily tilted vertically or horizontally by remotely shifting its feed.
To significantly reduce reflector weight, holes are punched in the surfaces and radomes, as shown in Figure 1. Such gridded antennas are not significantly affected by strong winds, and they require simple lightweight towers. Also, the reflectors can be remotely folded and deployed, an advantage for space applications.7
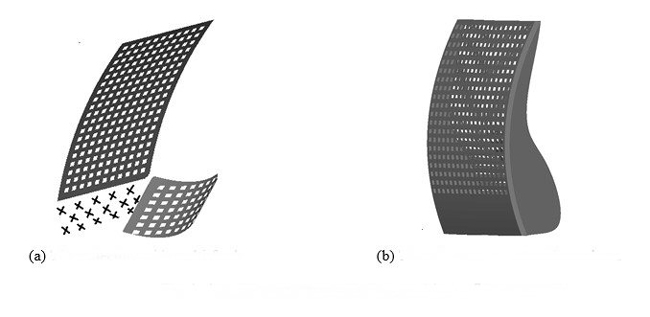
Figure 1 Multi-beam dual parabolic reflector antenna: reflectors with multi-feeds (a) and antenna covered with a radome (b).
COVERING THE AZIMUTH PLANE
The switched-beam smart base station antenna increases the number of beams covering the same main sector, which increases directional gain and reduces beamwidth. Hence, every user is near the peak of a beam. This is achieved in different forms, depending on the required number of beams, their vertical/horizontal tilt angles and required beamwidths. The azimuth plane is divided into either three 120 degree or four 90 degree main sectors (see Figure 2). Every main sector is covered by one of the sub-6 GHz multi-beam antenna units. Each unit can generate three, four, five or more beams (subsectors) within each main sector.
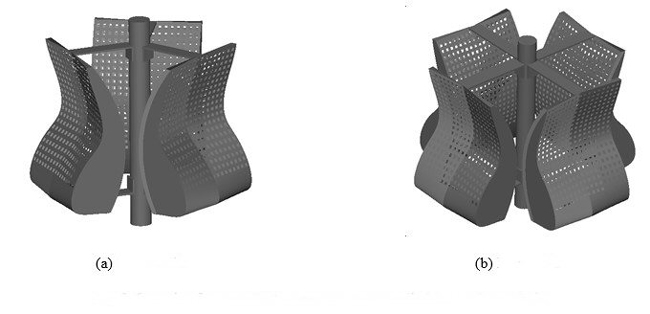
Figure 2 Three (a) and four (b) dual parabolic reflector units covering 360 degrees.
A sub-6 GHz multi-beam antenna is manufactured by a 3D printer, and the reflecting faces are covered by a thin layer of aluminum foil tape. The length and width of the main reflector are 80 and 37 cm, respectively, and the length and width of the subreflector are 17 and 40 cm, respectively. The focal lengths of the main and subreflectors are 40 and 20 cm, respectively. Several holes are punched in the antenna and the radome to reduce wind loading and weight. As the overall weight of each multi-beam unit with the radome is around 2 kg, the overall weight of the switched-beam antenna covering 360 degrees in azimuth with three or four units is about 6 or 8 kg, respectively.
The number of beams generated by each unit is controlled by the number of feeds and their locations. For example, three triple-beam units can be used, where each covers 120 degrees (see Figure 2a). With three triple-beam antennas, nine beams with 18 ports (±45 degree polarization) are generated to cover the entire azimuth plane at 4 GHz, as shown in Figure 3a. To further increase the overall number of beams, three quad-beam antenna units are used, where each covers 120 degrees. With three quad-beam antennas, 12 beams with 24 ports cover the entire azimuth plane, as shown in Figure 3b.
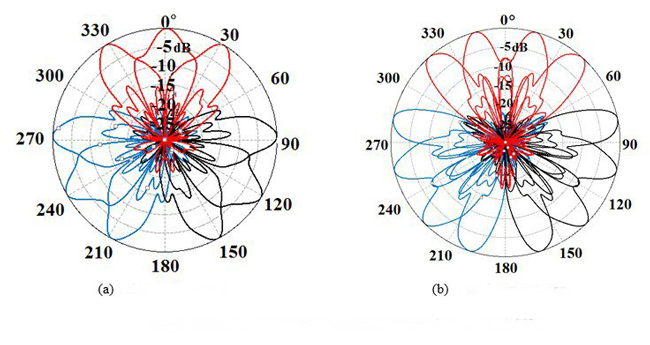
Figure 3 Radiation patterns of three antennas covering 360 degrees at 4 GHz: tri-beam (a) and quad-beam (b).
Two approaches used together can significantly increase the overall number of the horizontally sectorized beams covering the azimuth plane: The number of azimuth main sectors is increased from three to four, such that each covers a smaller azimuth angle (i.e., 90 instead of 120 degrees), as was shown in Figure 2b. Also, each main sector is covered by a larger number of subsectors (i.e., multi-beams). For example, four penta-beam antenna units are used, where each covers 90 degrees with five beams in each 90 degree azimuth main sector. With four penta-beam antenna units, 20 beams with 40 ports (±45 degree polarization) are generated to cover the azimuth plane at 4 GHz (see Figure 4).
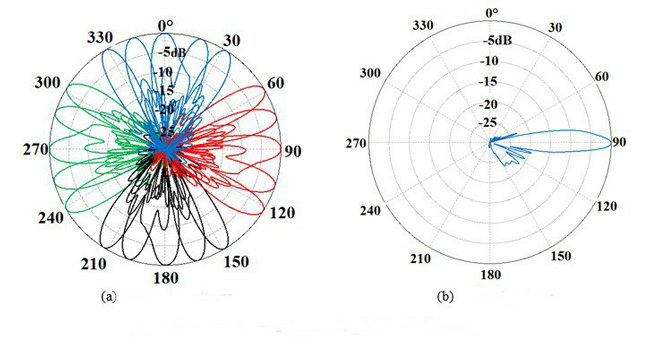
Figure 4 Horizontal (a) and vertical (b) patterns of four penta-beam units covering 360 degrees with 20 beams.
The radiation patterns of the above configurations (triple, quad and penta) and return losses are calculated using GTD® software, with the calculated results verified experimintally using a Rohde & Schwarz ZVL vector network analyzer and two calibrated reference feed horns on the roof of a high building (see Figure 5).
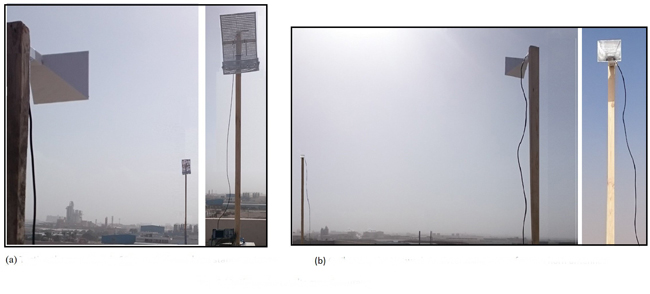
Figure 5 Experimental verification of the sub-6 GHz multi-beam base station antenna (a) and calibration using two reference horns (b).
The |S11| of the penta-beam configuration is shown in Figure 6. In all configurations (triple, quad and penta), the return loss is better than 14 dB over the frequency band from 3.3 to 7.0 GHz. The gain ranges from 20 to 24 dBi, and the front-to-back ratio at 180 degrees is greater than 30 dB. The first upper sidelobe suppression is 15 dB, and the isolation between polarizations is greater than 20 dB. Isolation between beams is greater than 21 dB. Each beam can be electrically tilted from 0 to –10 degrees.
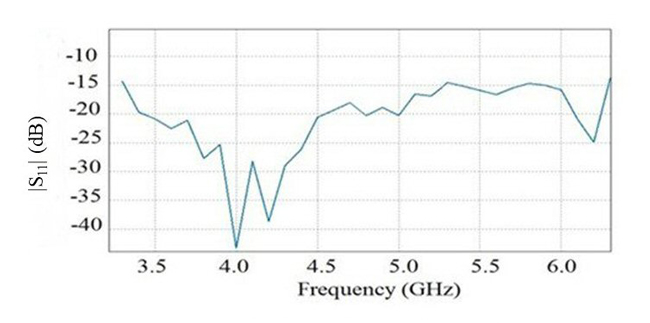
Figure 6 |S11| of the penta-beam antenna.