In 2019, 5G went mainstream. The first commercial deployments were rolled out, and mobile users in select markets began to get the first taste of 5G technology. Looking at the Gartner Hype Cycle (see Figure 1), 5G is at the peak of inflated expectations and should reach a plateau of productivity in two to five years. After the peak of inflated expectations, a period of disillusionment is anticipated, and it seems 5G will be no exception. The promises that 5G made cover numerous applications with numerous technological challenges. The first 3GPP release to cover 5G, release 15, only covered a small subset of the technology needed to fully realize the key performance indicators (KPI) of 5G: enhanced mobile broadband (eMBB), massive machine type communications (mMTC) and ultra-reliable low latency communications (URLLC). Release 15 focuses primarily on eMBB; only the subsequent releases will address mMTC and URLLC, with substantial work to complete these efforts.

Figure 1 Gartner Hype Cycle, August 2019. Source: Gartner.
5G PHASE 2
When 5G was first defined, there was a recognition that it was more complex and ambitious than previous generations, and development was split into two phases. The first phase, corresponding to release 15, was intended to address a subset of features for the first commercial deployments in 2019. The second phase, release 16, was intended to be a follow up to “address all identified usecases (sic) and requirements,” according to a 2015 3GPP RAN document (see Figure 2). Trying to address all additional uses cases in release 16 has proven to be unrealistic; features and use cases are being pushed into release 17 and beyond.

Figure 2 5G phased plan. Source: 3GPP.
Some of the topics that release 16 is addressing include integrated access and backhaul, side link enhancements for V2X, and New Radio Unlicensed (NR-U). Some of the topics that have been pushed for consideration in release 17 include non-terrestrial networks, positioning enhancements and spectrum above 52.6 GHz. Completing study items and work items for mmWave frequencies above 52.6 GHz is one of the noticeable technologies pushed into future releases, compared to the plan several years ago. The reason for this is that mmWave is hard. It is hard from a technology perspective to address the challenges with building and testing mmWave products that function indoors, outdoors and while moving. It is hard from a business perspective to create the right financial models for deploying this expensive new technology.
PUSHING HIGHER AND HIGHER
Much work remains to make mmWave successful for large commercial deployments. Yet, there is a desire from some in the research community to continue exploring how and if higher frequencies can be used for wireless communications. The 3GPP has only documented plans to look into frequencies at 114 GHz and below, but frequencies around 140 and 300 GHz - even up to 1 THz - are being explored, technology that is likely 10 years or more from commercialization. These higher frequencies have some unique properties because of their shorter wavelengths compared to lower frequency mmWave signals. For example, THz signals can be used for sensing applications like air quality detection or to implement touchless gestures for interacting with machines. There are even wider swaths of bandwidth available in the THz range, so much higher data rates are theoretically possible. With wavelengths smaller than 1 mm, using THz frequencies for location and positioning could provide centimeter accuracy.
These are promising aspects of THz technology, but there are challenges as well. Transceivers covering these frequency ranges are expensive, and there are few products on the market. Figure 3 shows one example of hardware that is available to enable THz research: a software-defined testbed comprised of the mmWave Transceiver System (MTS) from National Instruments (NI) with up- and down-converters, or frequency extenders, from Virginia Diodes (VDI). Another challenge for THz is the significant signal loss, which is even greater than at mmWave frequencies. The distances for which THz communications will be useful needs to be assessed, in addition to how these signals behave in different channel environments. Limited channel models exist today, and more measurements are needed to create robust models. As THz research is at a similar phase as mmWave technology 10 to 15 years ago, it seems clear that THz will not be included in 5G, while it is a promising candidate for 6G.
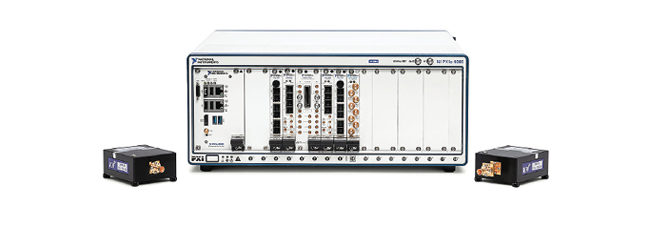
Figure 3 Combining a mmWave transceiver with extenders enables measurements from 110 to 170 GHz.
LOOKING FORWARD
Over the next five years, with any luck, 5G will move beyond the dreaded trough of disillusionment and into the plateau of productivity. For this to happen, mmWave research must continue. Beam tracking algorithms will improve. Chipsets will become more widely available and prices will begin to drop. As mmWave technology becomes more affordable, more researchers will have access to higher frequency equipment and applications like mmWave massive MIMO can be more widely explored. Breakthroughs in THz equipment, for research, commercialization and test, will also be needed to bring the technology out of research and solidify it as a 6G technology.
The next five years will be full of advancements in wireless communications. The list of use cases and applications 5G can support will expand, and the technologies that will shape 6G will become clearer.