

Harmonic load-pull systems have gained importance recently, because such setups allow the appropriate harmonic terminations for a transistor to be determined in order to obtain high efficiency and high linearity. Harmonic load-pull can also make the verification of transistor models possible. However, the active load-pull systems that are presently used are very complicated and present many difficulties in the measurements.1,2 An impedance tuner that transforms a 50 ? termination impedance into an arbitrary impedance can be realized with a 3 dB hybrid coupler and open or shorted stubs in its coupled and through ports. When the isolated port is terminated in 50 ?, the reflection coefficient at the input port is easily controlled by changing the stub lengths ?1 and ?2 in the coupled and the through port of the 3 dB hybrid, and can be expressed as3

The impedance tuner is used to measure the optimum input/output impedance of a microwave circuit such as an amplifier, mixer and so forth. Figure 1 shows a conventional impedance tuner using a 3 dB hybrid and shorted stubs for optimum input/output impedance transformation. A load-pull measurement is possible within the operating frequency band when the matching points for the device under test (DUT) are determined with impedance tuners, but the harmonic termination is almost impossible, due to the frequency characteristics of the 3 dB hybrid. Consequently, the operating conditions in the fundamental band can be changed due to the harmonics termination. A defected ground structure (DGS), which is realized by etching a dumbbell or spiral shaped pattern in the ground plane of a microstrip line, has been proposed.4 Several applications using DGS to design a coupler, a filter and a power amplifier have already been presented.5–8 Recently, a DGS microstrip line that can suppress the second and third harmonics by inserting an asymmetric defect in the ground plane has also been presented.9 The DGS microstrip basically provides a slow-wave effect by increasing the effective inductance of the transmission line.
In this article, an impedance tuner using a new 3 dB branch line hybrid is proposed. Since the proposed 3 dB branch line hybrid includes asymmetric DGS microstrip lines, the second and third harmonics can easily be suppressed. A ?/4 DGS bias microstrip is also used that effectively suppresses the third harmonic component as well as the second harmonic and low frequency intermodulation components.8–10 An amplifier harmonic load-pull setup can be easily implemented by using an impedance tuner and a ?/4 bias line that suppress the second and third harmonics.
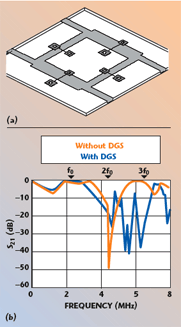
Harmonic Termination 3 dB Branch Line Hybrid Tuner
Although there are several shapes used for the defects of DGS microstrip lines, dumbbell or spiral shapes are the most common. The spiral DGS microstrip lines have steeper band-rejection characteristics than dumbbell DGS lines, but their band-rejection bandwidth is narrower. Several stage dumbbell DGS lines also have steep and wide band-rejection characteristics, but a larger circuit size is required than for the spiral. Therefore, an asymmetric spiral DGS that has a dual band-rejection characteristic at the specified frequencies is effective for circuit size reduction. Figure 2 shows the geometry of an asymmetric spiral DGS on the ground plane of the microstrip line, in which the dimensions of the spiral-shaped defects in the right- and the left-hand side are different (A = 3 mm, A' = 2.6 mm, B = 2.6 mm, B' = 2.2 mm, C = G = S = 2.2 mm, D = 2.4 mm). The transfer and the reflected characteristics are also shown. The substrate used is RT/duroid 5880 with a dielectric constant of 2.2 and a thickness of 31 mils. The circuit was simulated with HFSS from Ansoft. The asymmetric spiral DGS microstrip line is designed for the IMT-2000 base station transmitting band, and the transfer characteristic around the second and third harmonic bands is suppressed. A harmonic termination 3 dB branch line hybrid coupler, using asymmetric DGS microstrip lines, was simulated and fabricated. The fabricated hybrid is of a slightly smaller size than the conventional hybrid because of the slow-wave effect of the DGS microstrip lines. In a conventional 3 dB branch hybrid, the length and width of the 35.35 and 50 ? lines are 26.8, 3.9 and 26.4, 2.4 mm, respectively, but those of the proposed hybrid are 19.6, 5 and 25.6, 2.4 mm, respectively. Figure 3 shows the geometry of the proposed branch line coupler where the center frequency is 2.14 GHz. The coupling and the transmit coefficient of the 3 dB hybrid are –3.1 and –3.4 dB, respectively. Also shown is the transfer characteristic in the case when the open stubs at the coupled and through ports have the same electrical length. For comparison, the transfer characteristics of the conventional 3 dB hybrid are also shown. The transfer losses for the proposed hybrid, within the operating band and at the second and third harmonics, are –0.3, –15.5 and –28.9 dB, respectively. The measured results in the operating band and at the second harmonic show very similar characteristics to the conventional hybrid. But the characteristics at the third harmonic are quite different. It is definitely observed that the second and third harmonics cannot pass in the proposed harmonic termination 3 dB branch line hybrid.

Harmonic Blocking Bias Circuit
Small-signal amplifiers in the UHF band usually use chip inductors as RF chokes in their bias circuits. However, ?/4 long, high impedance transmission lines terminated with chip capacitors or radial stubs are usually used as bias lines at higher frequencies. When a capacitively terminated ?/4 bias line is connected to a signal line, even harmonic components that propagate on the signal line are blocked, while odd harmonic components pass through. In the signal amplifying process, an amplifier generates harmonics and intermodulation distortion components in addition to the amplified input signal. A proper harmonic termination is required to increase the efficiency and for active load-pull setup.
Figure 4 shows the layout of a harmonic blocking ?/4 bias line. Instead of a conventional microstrip line, a ?/4 DGS microstrip line is used. Due to the slow-wave effect of the DGS, the second passband is shifted down. As a result, the third harmonic of the amplifier can be reflected at the bias line junction point. A bypass LC series resonating circuit is also inserted in the bias pad of the ?/4 bias line, in addition to the bypass capacitor.10 This LC tank makes the low frequency blocking range broader. The transfer characteristics of the conventional and the proposed bias line are also shown for the low frequency band. The low frequency band-rejection of the proposed bias line is shifted down, so that low intermodulation components can be reduced compared to the conventional bias line. The transfer characteristics of the conventional and the proposed bias line in the operating and harmonic bands are also shown in the figure. The operating and second harmonic frequency characteristics are almost similar to that of the conventional bias line, but the third harmonic band is shift down, so that the second and third harmonics are blocked by the proposed bias line.
Amplifier Design Using Harmonic Termination Tuner and Harmonic Blocking Bias Line
To show the validity of the proposed harmonic termination impedance tuner and the harmonic blocking bias line, two kinds of amplifiers were fabricated and compared. One is realized with a conventional bias line and only in-band matching circuits. The other is made with the harmonic termination tuner and the harmonic blocking bias line. The operating frequency is 2.11 to 2.17 GHz and the transistor used is a FLL357ME device from Fujitsu.

Figure 5 shows the transfer and reflected characteristics of the conventional and proposed amplifiers. The measured gain, the maximum return loss and 1 dB compression point (P1dB) of the conventional amplifier are 13.03 ± 0.1 dB, –23 dB and 35 dBm, while those of the proposed amplifier are 13.38 ± 0.07 dB, –17.75 dB and 34 dBm, respectively. These characteristics show similar electrical characteristics in operating band except for P1dB. Because the impedance tuners were fabricated on PCB, the matching networks of the proposed amplifier were much bigger than the conventional. Therefore, if the impedance tuner could be fabricated on a high Q and high dielectric constant material, the P1dB of the proposed amplifier would be similar with that of the conventional one. Figure 6 shows the fundamental, the second and third harmonic characteristics for the two amplifiers. For the conventional amplifier, the second and third harmonics are 36.40 and 36.26 dB below the fundamental, while for the proposed amplifier they are 61.58 and 63.50 dB, respectively. It is evident that the second and third harmonic component levels in the proposed amplifier are much smaller than those of the conventional amplifier. This means that the proposed bias line and the proposed impedance tuner terminate the harmonic components properly.
Conclusion
In this article, a new harmonic termination impedance tuner using asymmetric DGS microstrips and a harmonic blocking ?/4 bias line are presented. These circuits suppress the harmonic components without affecting the electrical characteristics within the operating frequency band. By applying these circuits, a harmonic load-pull amplifier design can be easily performed. Since the fabricated harmonic termination impedance tuner was made with microstrip lines, its size is larger and its insertion loss higher. Consequently, the proposed amplifier has a smaller P1dB, compared with a conventional amplifier. If it was fabricated with low loss transmission lines, it would be possible to use it in a practical load-pull system. It is expected that the impedance tuner and bias line can be used in other microwave circuits.

References
1. R.B. Stancliff and D.D. Poulin, “Harmonic Load-pull,” 1979 IEEE International Microwave Symposium Digest, pp. 185–187.
2. J. Benedikt, R. Gaddi, P.J. Tasker and M. Goss, “High Power Time-domain Measurement System with Active Harmonic Load-pull for High Efficiency Base Station Amplifier Design,” IEEE Transactions on Microwave Theory and Techniques, Vol. 48, No. 12, December 2000, pp. 2617–2624.
3. Y.C. Jeong, “A Design of Predistortion Linearizer Controlling the Individual Order of Intermodulation,” Sogang University Dissertation, 1996.
4. C.S. Kim, J.S. Park, D. Ahn and J.B. Lim, “A Novel 1-D Periodic Defected Ground Structure for Planar Circuits,” IEEE Microwave and Guided Wave Letters, Vol. 10, April 2000, pp. 131–133.
5. J.S. Lim, C.S. Kim, J.S. Park, D. Ahn and S. Nam, “Design of 10 dB 90° Branch Line Coupler Using Microstrip Line with Defected Ground Structure,” Electronics Letters, Vol. 36, No. 21, October 2000, pp. 1784–1785.
6. D. Ahn, J.S. Park, C.S. Kim, J. Kim, Y. Qian and T. Itoh, “A Design of the Low Pass Filter Using the Novel Microstrip Defected Ground Structure,” IEEE Transactions on Microwave Theory and Techniques, Vol. 49, No. 1, January 2001, pp. 86–93.
7. J.S. Lim, C.S. Kim, D. Ahn, Y.C. Jeong and S.W. Nam, “Design of Low Pass Filters Using Defected Ground Structure,” IEEE Transactions on Microwave Theory and Techniques, Vol. 53, No. 8, August 2005, pp. 2539–2545.
8. Y.C. Jeong, S.G. Jeong, J.S. Lim and S.W. Nam, “A New Method to Suppress Harmonics Using ?/4 Bias Line Combined by Defected Ground Structure in Power Amplifier,” IEEE Microwave and Wireless Component Letters, Vol. 13, No. 12, December 2003, pp. 538–540.
9. D. Woo and T. Lee, “Suppression of Harmonics in Wilkinson Power Divider by Using Asymmetric Spiral DGS,” 2004 European Microwave Conference Proceedings, Vol. 1, pp. 467–470.
10. K. Kimura and M. Seki, “Improvement in ADLR Asymmetry for W-CDMA InGaP/ GaAs HBT Power Amplifier,” 2004 European Microwave Conference Proceedings, Vol. 1, pp. 333–336.