Industrial IoT Networks Powered by 5G New Radio
The fourth industrial revolution (Industry 4.0) triggered by industrial IoT (IIoT) will offer significant revenue expansion through new digital service provider markets. The main technology enablers for the IIoT revolution will comprise of 1.) 5G wireless converged automation protocols which will eliminate wires and support time synchronous operations, 2.) private edge cloud for secure and scalable local computing, 3.) deep slicing for IIoT networks so that multiple stakeholders can be supported using one infrastructure and 4.) machine learning (ML) enabled automated operations for monitoring, prediction and optimization of IIoT networks. Further, the IIoT network will be scalable in connectivity and number of devices and will be designed to deliver optimal performance for all industrial applications using key LTE and 5G New Radio (NR) features like ultra-reliable low-latency communication (URLLC), massive machine type communication (mMTC), 5G positioning, time sensitive communications (TSC) and, to a lesser degree, enhanced mobile broadband (eMBB).
In this article, we will begin with overview, use cases and requirements for IIoT. Next, we will discuss the foundation for URLLC in NR which was laid out in 3GPP Rel-15, mainly in support of IIoT. We then will address several IIoT related features in Rel-16 like URLLC enhancements, NR positioning and TSC and a version of NR known as NR-Light, which is planned to be introduced in Rel-17. NR-Light aims to address use cases that cannot be met by NR eMBB, URLLC or mMTC. Finally, we will present system performance for an indoor ray traced factory using key 5G NR features like URLLC and TSC.
IIoT powered by 5G networks will enable new value creation and offer significant revenue expansion for original equipment manufacturers (OEM) and network operators. IIoT will offer increased efficiency with industrial automation on a shared highly flexible virtualized and scalable infrastructure. It will be driven by key 5G NR features like URLLC, 5G positioning, TSC and, to a lesser degree, eMBB. IIoT will also be complemented by MTC technologies developed for LTE and known as enhanced MTC (eMTC) and narrowband IoT (NB-IoT). The foundation for URLLC was laid out in Rel-15 3GPP specifications. IIoT networks will be further enhanced by subsequent releases of 3GPP specifications (Rel-16 and Rel-17) with new features as outlined here.1-2
IIoT Use Cases and Requirements
A pictorial representation of Industry 4.0 use cases with the corresponding 5G technology features is shown in Figure 1. The two major Industry 4.0 verticals are manufacturing and transport/logistics industry. For the factory of the future, productivity must be improved while also improving safety and cost efficiency. Processes must be automated, and production uptime maximized to minimize business interruption to gain more efficiency and competitiveness and value for investments. At the same time, carbon emissions need to be reduced and energy be saved. To satisfy the above goals, one will see mass personalization of products, artificial intelligence (AI) backed automated testing, monitoring and analytics for production resiliency and digital value platforms for fast re-configuration of supply chains. The goal of the future transport/logistics industry is to increase throughput using existing infrastructure, eliminate consignment losses and transit time delays and ensure resiliency of IT infrastructure and control systems. To that end, transportation/logistics industry will see massive use of AI managed automated guided vehicles (AGV) for maximum throughput, resilient and secure control systems and massive instrumentation to ensure minimal carbon footprint.
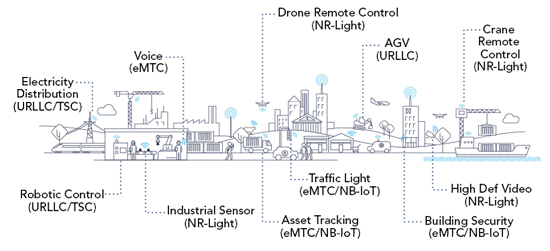
Figure 1 Industry 4.0 use cases and corresponding 5G NR features.
5G NR enables new value creation for Industry 4.0 based on three major use case domains, namely eMBB, URLLC and mMTC. Table 1 shows the requirements in terms of reliability, latency, data rate and connection density for a typical factory complex. Additionally, it shows the various 5G component technologies needed to meet the requirements for the use cases.
The beginning of Industry 4.0 is being showcased in Nokia’s 5G “factory of the future” in Oulu, Finland.3 This pre-production facility uses Nokia’s private wireless networks and edge cloud to securely connect all assets within and outside the factory.
Foundation for URLLC
Two key requirements were defined by 3GPP for URLLC use cases:4 1.) target user plane latency of 0.5 ms in the downlink and 0.5 ms in the uplink and 2.) packet reliability of 99.999 percent for a 32-byte packet with a user plane latency of 1 ms. The coupling of very high-reliability together with very low-latency makes URLLC requirements very challenging to satisfy. However, as noted in Table 1, many IIoT use cases do not require both ultra-reliability and low latency. To this end, 3GPP Rel-15 has separately specified two physical layer (PHY) feature groups to support URLLC.
The first group of PHY features are used to reduce latency. They include:
Flexible slot structure:
- Support of multiple subcarrier spacing values - NR supports subcarrier spacing of 15, 30, 60, 120 and 240 kHz. This translates into transmission slot length of 1, 0.5, 0.25, 0.125 and 0.0625 ms, respectively. Having a shorter slot length helps reduce latency as the data transmission process can be completed sooner. The disadvantage is that a less amount of data can be transmitted per slot. However, this is not crucial as URLLC data packets are typically small compared to e.g., eMBB.
- Sub-slot data transmission - NR allows data transmission that occupies less than a slot (i.e., 2, 4, 7 symbols in downlink, and any number of symbols in uplink). This allows data transmission to be completed in less time. For instance, with a subcarrier spacing of 60 kHz and 2 symbols, a packet can be transmitted in 0.036 ms.
- Self-contained slot structure in TDD - NR allows a slot in TDD to contain symbols for both data and hybrid automatic repeat request (HARQ) acknowledgment feedback. This reduces the wait time in TDD for uplink and downlink slots.
Flexible scheduling:
- Preemption - The base station can pre-empt on-going downlink data transmission to insert URLLC data into the data packet being transmitted. This is done by puncturing the on-going data transmission with URLLC traffic. The pre-empted UE is then notified of the pre-emption so it can appropriately manage the missing data.
- Configured grant or grant-free uplink transmission - User equipment (UE) are configured with periodic resources for uplink transmission. As a result, the UE does not have to transmit a scheduling request to the gNB (i.e., 5G NR base station) and wait for a scheduling grant whenever it has pending data.
Fast feedback:
- Shorter UE processing time - The NR slot structure design allows for UE pipelining to shorten the UE processing time.
- Short HARQ feedback format - A UE can transmit HARQ feedback in one or two symbols.
- Short periodicity for scheduling request - A UE can be configured with a scheduling request slot to request uplink data transmission as often as every 2 symbols.
The second group of PHY features are used to increase reliability. They include:
Data enhancements:
- Transmission of the same data packet from different transmission points to increase reliability. This is supported by duplicating the data packet at the packet data convergence protocol layer. It can be used, for example, to transmit the same packets from two different gNBs to the UE to increase reliability.
- Multi-slot repetition for data channels - Data packet can be transmitted using 2, 4 and 8 repetitions to improve reliability.
- URLLC-specific modulation and coding values that are designed to support very high-reliability.
Control enhancements:
- Support lower coding rate for the associated control channel and smaller control packet size.
- Enhanced channel state information reporting targeting 99.999 percent reliability.
In addition to the physical layer enhancements, URLLC also requires optimized system architecture design. Two important features are:
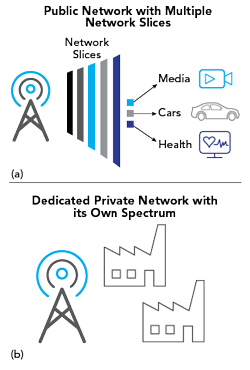
Figure 2 Two options for industrial networks.
- Edge cloud and multi-access edge computing (MEC) - Instead of a centralized architecture, an edge cloud places computing resources at the edge of the network. This allows for reduced end-to-end latency and ultra-fast response time. In addition, MEC is crucial to many IIoT verticals as it allows real-time data analytics and reduces the amount of data that must be sent upstream.
- Network slicing allows for a dedicated set of end-to-end resources to be allocated for multiple applications. For industrial networks, one option is to have public networks with slicing where each slice has guaranteed QoS and security level. The other option is to have a dedicated private network with its own “local spectrum” and customized security and QoS levels. These two options are illustrated in Figure 2.
IIoT Features in Rel-16 and Rel-17
In Rel-16, further enhancements for IIoT are being standardized in two work items. The first work item addresses physical layer enhancements for URLLC and includes the following items:5
- Control channel enhancements - Control format with configurable size to improve reliability, increased control channel monitoring capabilities to reduce scheduling delay, transmission of more than one acknowledgment within a slot and support for two simultaneous HARQ codebooks.
- Data channel enhancements - Mini-slot level hopping.
- Scheduling enhancements - Support for out-of-order scheduling and HARQ feedback, multiple active grant-free configurations.
- Uplink transmission prioritization and multiplexing among users - Interference cancellation to support uplink pre-emption and enhanced power control.
The second work item addresses support for new time-sensitive network use cases such as factory automation and electrical power distribution. These use cases have tighter requirements than URLLC (e.g., reliability of up to 99.999999 percent) and may also require strict time synchronization for packet delivery (e.g., deterministic services with bounded latency and extremely low variation). The work item includes the following enhancements:6
- TSC - Specify accurate reference timing delivery, address support for TSC messages, hard guarantees for QoS characteristics such as packet loss and reliability, latency bounds and synchronization down to the nanosecond level.
- Support PDCP duplication with higher reliability and better efficiency.
- Address resource conflicts and collisions between multiple transmissions from the same user.
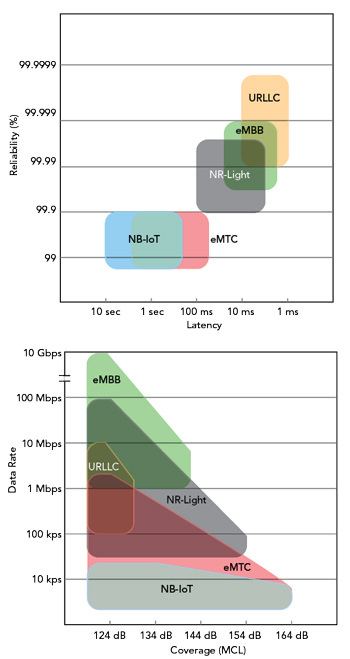
Figure 3 Comparison of NR-Light to other NR features.
In addition to the above work items, positioning is also an important aspect for IIoT deployment. In Rel-16, 3GPP is working to specify positioning support for NR.7 They include:
- Define interfaces, signaling and procedures.
- Extension of LTE Positioning Protocol (LPP) and NR Positioning Protocol “a” (NRPPa) for NR positioning.
- Specifying reference signals to support various positioning techniques.
- Define UE and gNB measurements and requirements applicable for positioning.
- Study the feasibility of RAN-based location management.
The positioning requirements for commercial use cases are:8
- Indoor deployment scenarios - Horizontal positioning error less than 3 m and vertical positioning error less than 3 m for 80 percent of UEs.
- Outdoor deployment scenarios - Horizontal positioning error less than 10 m and vertical positioning error less than 3 m for 80 percent of UEs.
- End to end latency less than 1 second.
Further, there is a need to address use cases that cannot be met by NR eMBB, URLLC, eMTC and NB-IoT. As such, a version of NR known as NR-Light will be introduced in the 3GPP Rel-17/18 time frame. With this new feature, operators can migrate their spectrum to NR which can support both URLLC and NR-Light on the same carrier as well as deploy eMTC/NB-IoT either in-band or in guard-band. The NR-Light comparison to other IoT related technologies with respect to data rates, latency and reliability are shown in Figure 3.
System Performance for a Typical Indoor Factory
In this section, we present simulation results for a typical indoor factory using ray tracing. It may be noted that, various real-time and ray tracing measurements in an industrial environment were done by multiple entities and used to derive IIoT channel model in 3GPP standards body.9-10
We chose to study performance using an indoor factory scenario with a 400 MHz bandwidth mmWave carrier at a 28 GHz carrier frequency. The high carrier bandwidths available in mmWave spectrum is very attractive from a capacity point-of-view, and the indoor factory setting inherently provides good isolation against the external environment which allows the possibility of using both licensed and unlicensed high bandwidth mmWave spectrum.
In this study we created a fictitious factory of dimensions 120 m x 50 m x 10 m with 12 single sector gNBs mounted on the ceiling and pointed downward. This is very similar to the indoor office scenario in the 3GPP TR 38.901, “Study on Channel Model for Frequencies from 0.5 to 100 GHz,” with the ceiling height increased from 3 to 10 m to be more appropriate of a factory setting.11 As for the propagation modeling, 3D ray tracing was utilized with the WinProp tool from Altair. Random rectangular metal objects were placed in the factory to be representative of production lines, heavy duty machinery, tool containers, etc. The walls and roof material were chosen to be concrete and metal support beams for the ceiling were added. This is depicted in Figure 4.
We chose to study the TSC use case as it is more demanding than looking at URLLC only. On top of the high-reliability with low latency (i.e., 99.999 percent reliability at less than 1 ms latency) as with URLLC only, TSC now also enforces that the delay between the transmitter and receiver is deterministic rather than variable. As such, a de-jitter buffer is added at the receiver to account for the variability in delay that occurs over the 5G NR air interface from things such as HARQ or variable scheduling delay that is seen with dynamic packet schedulers.
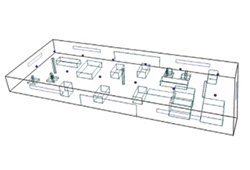
Figure 4 Fictitious factory floor which was ray traced for system simulations with 12 single gNBs placed on the ceiling in two rows with a spacing of 20 m between each gNB, horizontally and vertically.
The subcarrier spacing of 120 kHz used in the mmWave frequency band provides a short slot length of 0.125 ms. However, with a 1 ms latency constraint, this means only 1/0.125 = 8 slots are available for scheduling, and this must be split between uplink and downlink as mmWave spectrum is time-division duplex (TDD). For the symmetric traffic expected for the TSC use case, this means four slots are available per link direction, and this means only four analog beams could be configured to serve the entire gNB coverage area. This is typically an insufficient beamforming gain for mmWave spectrum, so instead it is advantageous to configure mini slots even for the 120 kHz subcarrier spacing. By choosing a 2-symbol mini-slot, which has a duration of just 17.84 μs, we now have 56 mini-slots in a 1 ms interval, leaving 28 mini-slots available per link direction when considering an equal uplink/downlink split when considering the symmetric nature of TSC traffic. Hence up to 28 analog beams can be configured in the system and sweeping over 28 beams in both uplink and downlink can be done in less than 1 ms.
The full set of system simulation assumptions are given in Table 2. The complementary cumulative distribution function of packet delay (CCDF) is given in Figure 5. In this case there were no reported packet failures. It is seen that the target of 99.999 percent reliability is achieved with less than 1 ms latency for both uplink and downlink. In Figure 6 we focus on just the downlink and increase the total number of active users in the factory. We look at 120, 300 and 600 active users, corresponding to an average of 10, 25 and 50 users per gNB, respectively. We see that the target 99.999 percent reliability level with less than 1 ms latency can be met even at these higher traffic loading, thanks to the wide bandwidth available in mmWave spectrum.
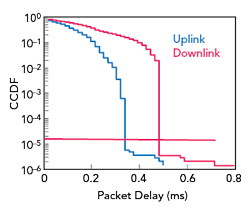
Figure 5 CCDF of packet delay for both uplink and downlink.
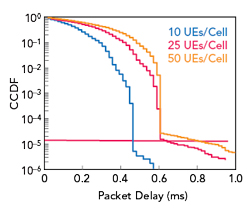
Figure 6 CCDF of packet delay in the downlink, with UE density of 10, 25 and 50 users per gNB corresponding to 120, 300 and 600 active users on the factory floor.
Conclusion
Industry 4.0 powered by 5G networks will offer significant revenue expansion through new digital service provider markets. 5G NR and its evolutions offer features like URLLC and its enhancements, TSC and its enhancements, network slicing and positioning, which are essential for IIoT deployments in both public and private networks. Simulation studies for a typical factory environment shows strict latency and reliability bounds can be met using 5G NR URLLC and TSC features.
Acknowledgments
The authors would like to thank Eugene Visotsky, Peter Merz and Andreas Maeder for their extensive help in preparing the paper.
References
- A. Ghosh et. al., “5G Evolution: A View on 5G Cellular Technology Beyond 3GPP Release 15,” IEEE Access, September 2019.
- 3GPP, “RP-190831; Key Directions for Release 17,” RAN#84, June 2019.
- D. Greenfield, “Nokia Employs 5G in its Own Factory,” AutomationWorld, August 12, 2019, www.automationworld.com/article/industry-type/discrete-manufacturing/nokia-employs-5g-its-own-factory.
- 3GPP TR 38.912, “Study on Scenarios and Requirements for Next Generation Access Technologies,” V14.3.0, June 2017.
- 3GPP, “RP-191584; Revised WID: Physical Layer Enhancements for NR Ultra-Reliable and Low Latency Communication (URLLC),” RAN#84, June 2019.
- 3GPP, “RP-191561; Revised WID: Support of NR Industrial Internet of Things (IoT),” RAN#84, June 2019.
- 3GPP, “RP-191156; Revised WID: NR Positioning Support,” RAN#84, June 2019.
- 3GPP TR 38.855, “Study on NR Positioning Support,” V16.0.0, March 2019.
- 3GPP, “R1-1909706; List of Measurements Used to Derive IIoT Channel Model,” RAN1#98, August 2019.
- 3GPP, “R1-1909807; Addition of Industrial IoT Model to 38.901,” RAN1#98, August 2019.
- 3GPP TR 38.901, “Study on Channel Model for Frequencies from 0.5 to 100 GHz,” V14.3.0, December 2017.